Translate this page into:
Molecular genetic changes in gastric carcinoma
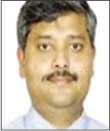
*Corresponding author: Puneet Kumar, Department of General Surgery, Institute of Medical Science, Banaras Hindu University, Varanasi, Uttar Pradesh, India. puneetimsbhu@gmail.com
-
Received: ,
Accepted: ,
How to cite this article: Singh J, Kumar P, Verma K, Tiwary SK, Narayan G, Dixit VK. Molecular genetic changes in gastric carcinoma. Int J Mol Immuno Oncol 2021;6(1):30-46.
Abstract
Gastric cancer remains highly prevalent and accounts for a notable proportion of global cancer mortality and this is associated with poor survival rates. Understanding the molecular genetic changes of gastric carcinoma may offer an insight into its pathogenesis helps in identifying new biomarkers, aid prognostication, and novel treatment targets. Over a past few decades, advances in technology and high throughput analysis have improved understanding of the molecular genetic aspects of gastric cancer. In this article, hierarchy of the changes at genetic and molecular level including several aspects which are heterogenous and represents a wide spectrum such as tumor suppressor genes, oncogenes, cellcycle regulators, apoptosis, cell-adhesion molecules, loss of heterozygosity, microsatellite instability, and epigenetic changes. The classification of gastric carcinoma at molecular and genetic level as well as hereditary gastric carcinoma is elaborated. The molecular genetic aspects regarding pathogenesis, changes and aberrations of all genes and pathways which are involved in gastric cancer are addressed in this review.
Keywords
Gastric carcinoma
Hereditary and acquired
Tumor suppressor genes
Oncogenes
MSI epigenetics
INTRODUCTION
Gastric cancer (GC) remains a worldwide burden as one of the leading causes of cancer-related death in both sexes. The late onset of clinical symptoms is the main reason that the disease is often diagnosed at an advanced stage, which limits the available therapeutic approaches in more than 50% of cases.[1] At present, GC is a most frequent diagnosed neoplasm worldwide. Although extensive studies have been performed to identify genetic pathways and genes involved in the disease development and progression, the prognosis of GC patients remains poor and little improvement of long-term survival has been achieved. Adenocarcinoma is the major histological type of GC, accounting for 90–95% of all gastric malignancies. The incidence is closely related to environmental factors, reflecting a characteristics geographical distribution.[2]
GC is a result of complex interaction of environment and multiple genes. The evident risk factors of GC include dietary, the Helicobacter pylori infection, the family history, and the genetic factors.[2,3] Although, there is great progression in the diagnosis and treatment of GC, the survival rate is still stagnant and poor, only about 20% of patients with GC can reach 5-year survival. Thus, a systematic view of the genetic basis of GC is necessary to establish new strategies for prevention and treatment of GC. Genetic factors mainly refer to the susceptible genes of cancer that is involved in multiple genetic and epigenetic alterations of oncogenes, tumor suppressor genes (TSGs), cell cycle regulators, DNA repair genes, and signaling molecules.[4]
GC is the second cause of cancer mortality[5] and the fifth most common malignancy in the world, 50% of the cases are from Eastern Asia[1] where China has the highest incidence.[6] The 5-year survival rate is still remain disappointing despite improvements in the diagnosis and treatment of GC as they are usually diagnosed at the advanced stage and usually incurable or rarely curable. The environmental risk factors have been established includes H. pylori infection, smoking, consumption of food high in salt, or N-nitroso compounds such as processed or smoked meats and Epstein–Barr virus (EBV) infection. The GC incidence seems to be increasing in younger age groups; however, the cause of phenomenon is still unknown.[7] Based on the Lauren classification, GC is classified as intestinal and diffuse types, which has different clinicopathological and prognostic features. It not only differs in morphology but also in epidemiology, progression pattern, genetics, and clinical pattern. Recently, it is seen that location of the tumor also matters as there appears to be a difference between proximal and distal non-diffuse GCs because of the distinct or different sets of gene expression level.[8,9] Surgical treatment is the only therapeutic modality which provides the greatest possibility of cure. Overall, this article aims to cover the molecular and genetic changes, different classification based on several basis genetic and epigenetic changes in GC which are currently understood.
CLASSIFICATION OF GASTRIC CARCINOMA
Early gastric carcinoma isclassified based on gross pattern as follows: Type I for the tumor with protruding growth, Type II with superficial growth, TypeIII with excavating growth, and Type IV for infiltrating growth with lateral spreading. Type II is again divided into IIa (elevated), IIb (flat), and IIIc (depressed) proposed by the Japanese endoscopic society.[10] Another classification, three gross patterns for superficial neoplastic lesions in gastrointestinal tract, the tumor is classified as: Type 0-I for polypoid growth (subcategorized to 0-IP for pedunculated growth and 0-IS for sessile growth), Type 0-II for non-polypoid growth (subcategorized into Type 0-IIa for slightly elevated growth, Type 0-IIb for flat growth, and Type 0-IIc for slight depressed growth), and Type 0-III for excavated growth.[11] The difference between intramucosal carcinoma and carcinoma insitu or high grade dysplasia is very important because the stomach intramucosal unlike the colon intramucosal shows metastasis. The useful important histologic features of intramucosal invasion are single tumor cell in lamina propria and significantly fused neoplastic gland of multiple sizes. The early gastric carcinoma prognosis is excellent and shows 5 years survival rate with about 90%.[12]
Advanced gastric carcinoma shows invasion upto muscularis propria beyond and has showed worse prognosis with 5-year survival rate at about 60% or less.[13] The gross appearance can be exophytic, ulcerated, infilterative, or combined. Based on Borrmann’s classification, the gross appearance is classified into; Type I for polypoid growth, Type II for fungating growth, Type III for ulcerating growth, and Type IV for diffusely infiltrating growth (linitis plastic in signet ring cell carcinoma), this happens when most of the gastric wall is involved by infiltrating tumor cells. In addition, World Health Organization (WHO) classification endorses other uncommon histologic variants, such as adenosquamous carcinoma, squamous carcinoma, hepatoid carcinoma, carcinoma with lymphoid stroma, choriocarcinoma, parietal cell carcinoma, malignant rhabdoid tumor, mucoepidermoid carcinoma, mixed adeno-neuroendocrine carcinoma, endodermal sinus-tumor, embryonal carcinoma, pure gastric yolk sac tumor and oncocytic adenocarcinoma, and micropapillary carcinoma of stomach is a newly recognized histologic variants characterized by small papillary clusters.[13]
CLASSIFICATION OF GASTRIC CARCINOMA BASED ON GENETIC PROFILE
According to Laurens classification, gastric adenocarcinoma isdivided into intestinal, diffuse, mixed, and indeterminate subtypes.[14] They vary not only in morphology but also in epidemiology, progression pattern, genetics, and clinical pictures. Histopathologicaly, intestinal type is characterized by malignant epithelial cells that show cohesiveness and glandular differentiation infiltrating the surrounding tissues.[15] In contrast, the diffuse subtype is characterized by tumor cells that show poor differentiation and lack of cohesion. Intestinal type of GC is felt to be caused mainly by environmental (exogenous) factors whereas the diffuse type is thought to be due to hereditary and genetic (endogenous) factors. These histologic classifications are not sufficient to reflect the molecular and genetic characteristics of GC or to develop personalized treatment strategies in the era of precision medicine. Several molecular classification systems have been proposed, and distinct molecular subtypes have been identified.[16-18] In 2014, the cancer genome atlas (TCGA) proposed foursubtypes: (1) EBV-positive (8.8%), (2) microsatellite unstable/instability (MSI, 21.7%), (3) genomically stable (19.7%), and (4) chromosomally unstable/chromosomal instability (CIN, 49.8%).[16] Most EBV-positive tumors occurred in male patients and in the gastric fundus or body, displaying extreme DNA hypermethylation and amplification of JAK2 and PD-L1/2, with 80% harboring non-silent PIK3CA mutations. All EBV-positive GCs displayed CDKN2A promoter hypermethylation, while lacking the MLH hypermethylation characteristic of the MSI-associated CpG island methylator phenotype (CIMP).[16,19]
MSI-subtype tumors tends to occur in female patients, diagnosed at advanced stage, and characterized by elevated mutation rates, including mutations of genes encoding targetable oncogenic signaling proteins. The genomically stable sub-type lacked numerous molecular alterations, correlated well with the Lauren diffuse histologic variant, but harbored mutations of RHOA or fusions involving RHO-family guanosine triphosphatase (GTPase)–activating proteins. The active GTP-bound form of RHOA activates signal transducer and activator of transcription-3 (STAT-3) to promote tumorigenesis. Finally, CIN subtype tumors were frequent at the gastroesophageal junction/cardia, correlated well with the Lauren intestinal histologic variant, showed marked aneuploidy, and harbored focal amplifications of receptor tyrosine kinases (RTKs), in addition to recurrent TP53 mutations and RTK-RAS activation.[16]
In 2015, the Asian Cancer Research Group (ACRG) proposed a new classification system associated with distinct genomic alterations, disease progression, and prognosis across multiple GC cohorts.[17] On the basis of whole-genome sequencing, gene expression profiling, genome-wide copy number microarrays, and targeted gene sequencing, four molecular subtypes were identified: (1) MSI, (2) microsatellite stable with epithelial-to-mesenchymal transition features (MSS/EMT), (3) MSS/TP53 mutant (MSS/TP53þ), and (4) MSS/TP53 wild-type (MSS/TP53).[17] MSI tumors were hypermutated, intestinal, usually antral, and diagnosed at clinical Stage I/II. MSI tumors had the best prognosis; their recurrence rate after surgical resection of primary GC was the lowest among all four subtypes (22%). MSS/TP53þ tumors were linked to EBV infection and had the next best prognosis, followed by MSS/TP53- tumors. MSS/EMT tumors occurred at a younger age, mostly diagnosed at clinical Stage III/IV, and were the Lauren diffuse histologic type. The MSS/EMT subtype had the worst prognosis and the highest recurrence rate (63%), with recurrences located mostly in the peritoneal cavity.[17] To refine molecular classification, regular mutated and hyper mutated GC types were identified in another recent study. The regular mutated type was further sub classified into two subgroups, C1 and C2. The first subgroup, C1, was enriched in mutations of TP53, XIRP2, and APC and was associated with a significantly better prognostic outcome, whereas C2 was over represented by mutations in ARID1A, CDH1, PIK3CA, ERBB2, and RHOA.[17]
MOLECULAR PROFILE IN GASTRIC CARCINOMA
TCGA has characterized 295 cases of gastric adenocarcinoma, the most comprehensive study to date, using multiple high-throughput technologies, which includes somatic copy number analysis, DNA methylation profiling, messenger RNA, and micro-RNA sequencing, reverse phase protein array, microsatellite instability (MSI), and whole genome sequencing.[16] After this, four GC subtypes were described they are; (1) Tumor positive for EBV, (2) MSI-high tumors, (3) genomically stable tumors, and (4) tumor with chromosomal instability [Table 1]. Moreover, these GC subtypes showed different epigenetic changes and mutation of several genes like; EBV + tumors show recurrent PIK3CA and ARIDIA mutations, extreme DNA hypermethylation, and high amplification of JAK2, PD-L1, and PD-L2. MSI-H tumors show elevated mutation rates which also include mutation of genes encoding targetable oncogenic signaling proteins.[17] GS (genomically stable) tumors are enriched by the diffuse histological variants and CDH1 and RHOA mutation or CLDN18-ARCHGAP fusion. CIN tumors observed frequently at the gastroesophageal junction/ cardia along with recurrent TP53 mutation and number of amplifications of RTKs genes.
The cancer genome atlas classification | Defining characteristics |
---|---|
EBV+ | PIK3CA, ARID1A, TP53mutation |
CDKN2A silencing | |
PD-L1/L2 overexpression | |
CpG island methylator phenotype | |
Male predominance | |
Increased immune cell signalling | |
More frequently in fundus and body | |
MSI | Hypermutation |
TP53, KRAS, PIK3A, ARID1A mutation | |
CpG island methylator phenotype | |
MLH1 silencing | |
Mitotic pathways | |
Diagnosed at older age | |
Female predominance | |
GS | CDH1, RHOA mutations |
Increased expression of cell adhesion pathways | |
CLDN18-ARHGAP fusion | |
Diagnosed at earlier age | |
Diffuse histology | |
CIN | RTK-RASactivation |
Aneuploidy | |
TP53mutation | |
More frequently at gastroesophageal-junction and cardia | |
Intestinal histology |
The ACRG has proposed four molecular subtypes which are as follows; (1) MSH-H, (2) MSS/EMT, (3) MSS/TP53− mutants, and (4) MSS/TP53+ wild type [Table 2].[17] The recent molecular characterization of GC is evolving. Many molecular classifications being proposed and different molecular subtypes have been identified.[19] Various RTKs such as human epidermal growth factor (EGF) receptor 2, EGFR1, mesenchymal epithelial transition factor (MET), and fibroblast GF receptor 2 (FGFR2) being reported to be amplified in GC and target therapies have been developed.[20-23]According to GI-screen as the nationwide cancer genome screening project, the frequently detected mutations are TP53 (47.8%), PIK3CA (9.2%), KRAS (6.0%), SMAD 4 (5.1%), APC (4.1%), TET2 (3.9%), and ERBB2 (3.3%), and copy number variants are ERBB2 (11.3%), CCNEI (11.1%), KRAS (3.7%), FGFR2 (3.3%), ZNF217 (3.3%), MYC (2.7%), CCND1 (2.3%), and CDK6 (2.1%).[24]
Asian cancer research group classification | Defining characteristics |
---|---|
MSI | |
Primarily intestinal histology | |
Predominantly in antrum | |
Heavy mutational burden | |
Higher rate of liver-limited recurrence | |
Best overall survival, earliest stage at diagnosis | |
MSS/EMT | |
Worst overall survival, higher stage at diagnosis | |
Younger age | |
Primarily diffuse histology | |
Highest rate of recurrence, peritoneal spread | |
Lowest mutational burden | |
MSS/TP53+ | |
Second-best overall survival | |
Highest percentage EBV1 tumors | |
MSS/TP53- | |
Higher rate of liver-limited recurrence |
The understanding of the molecular aspects of GC is improved by next generation sequencing (NGS) studies which provides high throughput method to know and identify the genetic alteration in GC systematically. Li-Chang et al. showed mutations of several driver genes by performing NGS that includes; TP53, PIK3CA, CTNNB1, CDH1, SMAD4, and KRAS.[25] Few of the TSGssuch asAPC, CDH1, CDH4, THBS1, and UCHL1 are found to be inactivated by hypermethylation.[26-29] It has been shown that 59% of GCs shows mutation in chromatin remodeling genes such asARID1A, PBRM1, and SETD2.[30] There are new mutated driver genes found, they are (MUC6, CTNN2A, and GLI3) through whole genome sequencing.[31] It was also found that the genes involved in cell adhesion and chromosome organization showed frequent mutations in gastric adenocarcinoma patients by which it has confirmed that there are 30 candidate driver mutation mutated in primary and lymph node tissues and, on the other hand, seven candidate mutation mutated only in primary tumors. The primary tumors show more mutations than metastatic tumors because metastatic tumors comes only from the subpopulation of primary tumors but surprisingly researchers do not found any metastatic specific mutations and it is expected that these metastatic tumor cell shows the behavior of continuing the mutations of its genome to gain more growth power. There are some locus at chromosome 17q12 which are frequently amplified in GC and they are as follows; PPPIRIB-STARD3-T-CAP-PNMT, PERLD1-ERBB2-MAC14832-GRB7.[32]
In addition to this, there are two genes which are very important located on 9p21 chromosome which encodes P16 and P15, respectively, they are CDKN2A and CDKN2B showed CN loss (CN=0.8~1.32) as these two genes normally show very crucial function, they inhibit cyclin-dependent kinase (CDK4) and CDK6 and control cellular proliferation by preventing entry into the S phase of the cell cycle and so inactivation of these may leads to uncontrolled growth in cancer.[33]
GENETIC CHANGES IN GC
Mutation of genes in GC is broadly classified into three categories;
High frequency drivers which display high rate of recurrence (>5–10%) across multiple GCs
Low frequency drivers which are recurrently mutated in the 1–10% range but they are still contributing to pathogenesis of disease
Passenger mutation/bystander which arises as a consequences of underlying mutational processes such as CpG deamination but are not functionally contribute in tumorigenesis.[34]
Recently, it has been reported the importance of RTK/ RAS/mitogen-activated protein kinase (MAPK) signaling, frequent mutations in the ERBB3 RTK, and the ligand/RTK NRG1/ERBB4 genes in GCs. The recent NGS studies have been highlighted two new GC genes–ARID1A and RHOA. ARID1A is mutated in 10–15% of GCs which is known to encode a components of SWI/SNF chromatin remodellar complex.[34] Mutation of ARID1A are typically inactivating (frameshifts, and non-sense mutation). Consistently acting as a tumor suppressor genes. The normal function of ARID1A gene is a role in GC cell proliferation through the control of cell cycle regulators CCNE1 and E2F1. The another genes RHOA which has been recently shown that it exhibits recurrent mutations in diffuse type/genome stable GCs.[34]
The consequences of mutation in both ARID1A and RHOA are different. ARID1A mutation is dispersed throughout the genes, whereas the RHOA mutation is localized to an N-terminal hot-spot region (Ty42, Arg5and Gly17). ARID1A are predicted to modulate downstream Rho-signaling.
Mutation of RHOA suggests after functional studies that they can impart resistance to anoikis (a form of programmed cell death occurring after cellular detachment from a solid substrate). Clinically, RHOA hotspot mutation discovery provides specific inroad for the development of new approaches to target diffuse type GCs (DGCs), traditionally associated with extremely poor prognosis.[34] Genomic studies also uncovered the long-tail of low frequency driver mutations in GC such as gastric mucin MUC6, BCOR (encoding BCL6 corepressor), FAT 4 (a protocadherin), and RNF43, a Wnt pathway regulator. Although they have low mutation frequency, these genes also contributes to the ability of GC to manifest the cancer hallmarks.[34]
TSG
TSGs normally show protective role in preventing the malignant transformation of cells by repairing DNA inhibiting cell proliferation and initiating programmed cell death (apoptosis). TSGs are involved in the regulation of a range of cell functions including cell adhesion, cell-cell interaction, cytoplasmic signal transduction, and nuclear transcription.[35] Over recent decades, there is rapid expansion in the member of TSGs which have been identified in relation to a broad range of inherited and non-inherited human cancers. Greater understanding of the pattern of TSG expression in GC may enables the identification of specific biomarkers which could be used for early diagnosis and development of target treatment. The overexpression of P53 and loss of expression of phosphatase and tensin homolog (PTEN), E-cadherin, SMAD4, MGMT, and CD82 were all significantly associated with poor prognosis in gastric carcinomas.[36]
Tumor protein P53 (TP53): It is the most frequently mutated aberration in ~50% of GC cases. The cellular function of TP53 is a guardian of genomic integrity. The GCs in which TP53 gene is mutated often exhibit high levels of SCNAs involves both broad chromosomal regions and focal gene regions. The mutations in other canonical oncogenes (KRAS, CTNNB1, and PIK3CA) and tumor suppressor genes (SMAD4, APC) have also seen in GCs.[37] The aberrant expression of TP53 have been found in over half of human cancers including leukemia, breast, colon, and lung carcinoma and alteration in TP53 is the most common event in human cancers. Normally, TP53 plays key role in cell cycle progression preventing G1/S transition after DNA damage occurred and allowing DNA repair/cell apoptosis.[37] Some studies shown that TP53 abnormalities can occurs in nonneoplastic gastric mucosa with intestinal metaplasia suggesting TP53 mutation as early phenomena in gastric carcinogenesis[38]
PTEN: It is aTSG, discovered in 1997. It functions as a dual-specificity protein and phospholipid phosphatase, and regulates a variety of cellular processes and signal transduction pathways in a complex network system that can control proliferation, apoptosis, migration, adhesion, and genetic stability. The most detailed study of PTEN is focused on its negative regulation on phosphoinositide 3-kinase (PI3K)/Akt signaling pathway, which is important in controlling cell survival, promoting proliferation, and inhibiting apoptosis. It is a lipid phosphatase which phosphorylates phosphotidyl inositol-3,4,5-triphosphate (PIP3) reduces the activation of PI3K and the serin-threonine specific protein kinase Akt.[39]Hence, loss of expression of PTEN, increased level of PIP3, increase AKT activation, and consequently inhibits apoptosis which ultimately results in large number of tumors. There are multiple phenomena due to which PTEN inactivation occurs such as gene mutation, loss of heterozygosity (LOH), hypermethylation or miRNA-mediated alterations in gene expression, or post-translational modification.[40] In gastric adenocarcinoma, PTEN protein expression is significantly downregulated compared to normal. On the other hand, PI3K, AKT, MMP-2, MMP-9, and nuclear factor-κB (NF-κBp65) protein is overexpressed in GC[41]
CDH1 (Cadherin 1): Inactivation of E-cadherin found in early stage of gastric carcinogenesis, tumor progression, invasion, and metastasis. CDH1 gene alteration leads to loss of E-cadherin expression which ultimately results in cancer cell proliferation, invasion, and metastasis which is considered as primary carcinogenic event in hereditary diffuse GC.[42-44] Somatic alterations in CDH1 found in approximately 30% of GC patients. About one-third having structural alterations (7.5% LOH, 1.7% mutation) and rest having epigenetic modifications with 18.4% hypermethylation.[45] Epigenetic modification is more common than structural in diffuse type while intestinal type has approximately similar rates of structural and epigenetic changes.[45] Wei et al.[46] recently found that mRNA and protein expression level of T-cadherin is lower significantly in gastric tumor tissues compared to normal adjacent tissue.[46] The decreased T-cadherin expression is correlated with larger tumor size, lymph node metastasis, and higher TNM stage.[46] Multivariate analysis shows that T-cadherin expression is an independent prognostic factor for overall survival[46]
SMAD3 is another tumor suppressor gene whose loss of expression is associated to advanced stage and poorer outcome in gastric carcinoma patients which is more common in intestinal type than in diffuse type gastric carcinoma
SMAD4 loss of expression is more common in intestinal type while E-cadherin loss is more common in diffused type.[36] P53 expression loss is more common in poorly differentiated tumors.[36] Loss of E-cadherin, SMAD4, CD82, MGMT, and PTEN expression is associated with advanced stage of disease while P16 lossed expression seen in both early and advanced stages.[36] Loss of TSG expression accumulation in GC tumor expression which has significant correlation between this accumulation and patient survival.[36] Apart from mutation, genetic instability, activation of oncogene, and aberrant GF expression/receptor activation all together play role in gastric carcinogenesis
Retinoblastoma protein 1 (RB1) is involved in negative cell cycle regulation at the G1/S transition. RB1 shows LOH in advanced stage. RB1 is known to be a target of miRNA-106b~25 which is involved in promotion of cell proliferation, EMT, cell cycle progression, and exerts an apoptotic effect[47]
Pre-myelocytic leukemia is a TSG controls apoptosis and cell proliferation through P73 and yes associated protein-1 which are found downregulated in GC[47]
Fbxw7 is a TSG that is responsible for the degradation of several proto-oncogenes and its functional inactivation can dysregulate the cell division process, and potentially lead to tumorigenesis. The inactivation of Fbxw7 in the progress of human cancer including mutation, deletion, and hypermethylation, of which the Fbxw7 mutation is most common.[48] The Fbxw7 mutation rate in GC tissues was from 3.7% to 6% and did not differ in early or advanced GC, which might play a role in the prognosis of GC.[49] Fbxw7 mRNA expression level in cancerous tissues was lower than that in noncancerous tissues. The low Fbxw7 expression had remarkable poorer prognosis than those with high Fbxw7 expression.[50] Fbxw7 expression was associated with the progressive tumor size, lymph node metastasis, peritoneal dissemination, venous invasion, and clinical stage. Fbxw7 induced tumor apoptosis and growth arrest and inhibited the EMT in part by downregulating the RhoA signaling pathway in GC.[51]
Oncogenes
Oncogenes are genes whose normal activity promotes cell proliferation. Oncogenes function through the mechanism of gene mutation, gene insertion, chromosomal translocation, gene amplification, and DNA hypomethylation. Oncogenes can be classified into five broad classes: Secreted GFs; cell surface receptors; components of intracellular signal transduction systems; DNA-binding nuclear proteins; components of the network of cyclins, CDKs, and kinases inhibitors that govern progress through the cell cycle(s).[52]
Oncogene in humans hasthe capacity to transform normal cells into malignant ones. These genes are mutated during life make the patients more prone or susceptible to cancer by altering or impairing several phenomenon:[52] (1) Production of nuclear transcription factors (TFs) that control cell growth (e.g., myc), (2) signal transduction within cells (e.g., RAS), (3) interactions of GFs and their receptors (e.g., her/neu). Mutations convert proto-oncogenes into oncogene by several processes such as amplification, translocation, and point mutation. Oncogenes are activated by many ways through oncogene amplification, activation by point mutation, and activation by production of chimeric gene products.
RAS
The first human oncogene identified which is involved in 20% of all human malignancies. These are the oncogenes which are not present in normal cells rather they are activated in tumor cells as a consequences of enormous mutations occurs during tumor development. RAS gene encodes guanine-nucleotide binding proteins which perform various functions in mitogenic signal transduction and protein activity of RAS is controlled by GTP or GDP binding (active – GTP bound and inactive-GDP bound) states.
C-myc
It is an another oncogene which is located on chromosome 8 encodes a nuclear phosphoprotein which acts as TF whose main function is to regulate the transcription of target genes throughinducing and repressing the expression.[53] It also involves in modulation of several genes which are involved in key cellular processes including proliferation, growth, differentiation, and angiogenesis, but also DNA repair and apoptosis,[54] which if deregulated (myc gene) contributes to the formation/genesis of a wide variety of human solid tumors. Overexpression of C-myc is found in over 40% of GCs. It is an early event during the genesis of both intestinal and diffuse type of gastric carcinomas, but significantly higher expression in intestinal type than diffuse type. The C-myc overexpression is associated with poor survival. It has been found that in benign gastric lesions including chronic atrophic gastritis, gastric ulcer, and H. pylori infection, there is high expression of C-myc gene.[55] The another protooncogene FOS and JUN gets converted to oncogene isthe components of AP-1 complex acts as TF which activates transcription of several targets genes. The FOS and JUN oncogenic proteins are self-sufficient for cell transformation which also have very crucial role in gastric carcinomas.[56-58]
Prolin rich protein 11 (PRR11)
In 2013, PRR11 identified as novel gene which has been functionally characterized and discovered that, PRR11 plays important role in cell cycle progression and tumorigenesis and its protein has an oncogenic role in GC. The silencing of PRR11 in several gastric cell lines inhibited several proliferation rate, cancer cell migration, and cell colony formation and tumor growth invivo experiments.[59,60] The results showed that PRR11 mRNA and protein are upregulated in GC tissues as compared to normal gastric mucosa. PPR11 shows positive expression which was significantly associated with an aggressive cancer phenotypes including tumor with increased amount of invasion, increased tumor differentiation, and advanced disease stage.[61] However, knockdown of PRR11 in gastric cell lines showed diminished cellular proliferation and decreased colony formation which suggests that PRR11 protein expression plays critical oncogenic role in the development and progression of gastric carcinoma.[61]
Cell cycle regulators
Cyclins are proteins that control progression through key checkpoints in the cell cycle by binding and activating specific CDKs. From G1-S phase is regulated by the activities of the cyclin D, cyclin E, cyclin A, and their catalytic partners such as CDKs 2,4, and 6. The G2/M transition is regulated by B-type cyclin-associated kinase. The cyclin-CDK complexes stimulate the cellcycle progression and CDKIs induce the cell cycle arrest by downregulating CDK activity.[62] Moreover, the dysregulated expression of these cell cycle related molecules gives rise to uncontrolled proliferation and the malignant transformation of the cell.[63] Cell cycle control is governed by D-type cyclins which is most commonly mutated pathways in tumor cells. There are accumulating evidences that gastric carcinogenesis frequently involve abnormalities in the expression of cyclin and other cell cycle related genes.[64]
CyclinD1 (CCND1 gene): Located on chromosome 11q13 encodes members of G1 cyclin family which are involved in regulation of cell cycle and replication. Tumor specific alterations leads to deregulation of cyclin D1 which exhibit oncogenic potential in number of processes such as abnormal growth, angiogenesis, and resistance to apoptosis. Increased cyclin D1 expression has been shown in about 50% of primary gastric tumors. It is associated with signet ring phenotype and poor differentiation in few studies which show no pathologic features, no non-signet ring phenotype.[65] Cyclin D1 expression found in 50% of GCs, but more commonly in the intestinal type than in the diffuse type.[65]
Cyclin B1 is seldom studied in gastric tumor. The cyclin B1 expression and its correlation with clinicopathological features and the relationship of its expression to prognosis remain unclear until the present date.[66] The cyclins expression and negativity for CDK inhibitors are important mechanisms related to gastric carcinogenesis but only cyclins B1 expression is associated with reduced survival. Hence, it can be suggested that the expression of cycle B1 could be considered as a marker of aggressive biological behavior in GC.[66] The expression of cyclin B1 has been found to play important role in lymph node metastatic potential in GC.[66] Cyclin CDKs and CDKIs, tumor suppressor gene P53 and pRb alterations are frequently observed in human neoplasm, including gastric carcinomas.[67] The decreased expression of CDKI p27KIPI in GC is frequency associated with depth of tumor invasion and the presence of lymph node metastasis.[68] Cellular proliferation follows an organized and timely regulated progression through the cell cycle, which is controlled by protein complex composed of cyclins and CDKs.
Apoptosis
Apoptosis was initially described by its morphological characteristics, including cell shrinkage, membrane blebbing, chromatin condensation, and nuclear fragmentation.[69-71] The realization that apoptosis is a gene-directed program has had profound implications in understanding the developmental biology and tissue homeostasis, it implies that cell numbers can be regulated by factors that influence cell survival as well as those that control proliferation and differentiation. Moreover, the genetic basis for apoptosis implies that cell death, like any other metabolic or developmental program, can be disrupted by mutation. In fact, defects in apoptotic pathways are now thought to contribute to a number of human diseases, ranging from neurodegenerative disorders to malignancy.[72] What triggers apoptosis during tumor development? A variety of signals appear important. Extracellular (EC) triggers include growth/survival factor depletion, hypoxia, radiation, and loss of cell-matrix interactions. Internal imbalances can also trigger apoptosis, including DNA damage (produced by cellcycle checkpoint defects or exogenous toxins), telomere malfunction, and inappropriate proliferative signals produced by oncogenic mutations.
The cloning and characterization of the Bcl-2 oncogene established the importance of apoptosis in tumor development. Bcl-2 was first identified at the chromosomal breakpoint of t (14;18) in a human leukemia line.[73,74] To date, at least 15 Bcl-2 family member proteins have been identified in mammalian cells, including proteins that promote apoptosis and those that prevent apoptosis.[75] In addition to Bcl-2, Bcl-xL is a potent death suppressor that is upregulated in some tumor types.[76]In vitro studies have shown that both GKN1[77-81] and GKN2[81] occur in the gastric mucosa of healthy individuals. On the other hand, in the gastric mucosa of GC patients compared to subjects with superficial gastritis, a decreased GKN1 protein,[82] and mRNA GKN1 expression is noted.[83,84] GKN1 maintains the integrity of the gastric mucosa, protects it against the action of stomach acid, and enzymes as well as mechanical damage, bacteria or foreign antigens.[78,85] GKN1 has been shown to inhibit tumor cell growth and reduces the number of cell colonies by stopping the G2/M cell cycle instead of inducing apoptosis.[80] On the other hand, it was found that in the AGS GC cell line transfected with GKN1, inhibits proliferation of these cells and activation of the apoptosis process.[86]
Cell adhesion molecules
Classical cadherins are transmembrane-spanning adhesion molecules containing five calcium-dependent EC domains that confer homotypic interactions and a cytoplasmic tail that binds to a number of effectors to transduce physical and biochemical signals to the cell.
The names of cadherin were initially based on the cell type in which expression was first described, a consensus nomenclature now defines the classical cadherins as CDH1 (E-cadherin), CDH2 (N-cadherin), CDH3 (P-cadherin), CDH4 (R-cadherin), and CDH15 (M-cadherin).[87] Evidence for a direct function of E-cadherin in cell-cell adhesion was provided when the full-length cDNA of E-cadherin was cloned and expressed in fibroblasts that do normally not express E-cadherin. The pivotal role of E-cadherin during normal epithelial function might form the basis for its function as a tumor suppressor. E-cadherin-inactivating mutations in GC preferentially cause in-frame deletions caused by skipping of exons 7 or 9, or occasional frameshift mutations
E-cadherin expression is mostly restricted to epithelial cells, whereas cells of neural or mesenchymal origin usually express N-cadherin. Epithelial cells are phenotypically dissimilar from mesenchymal cells; from a cancer point of view the latter are more motile and migratory. Cadherin switching in cancer is defined as loss of E-cadherin and expression of N-cadherin during tumor progression,[88] which will induce or enhance the metastatic capacity of the invading carcinoma cell. Germline E-cadherin (CDH1) mutations are found exclusively in DGC.[89]
During EMT, Type I cadherin (epithelial-cadherin, E-cadherin, encoded by the CDH1 gene at human chromosome 16q22.1), which sustains key intracellular binding structures such asdesmosomes and claudins, is switched to neural cadherin (N-cadherin, encoded by the CDH2 gene), which is mostly expressed among mesenchymal cells.[90] The reduction of E-cadherin with the immunoglobulin-like domain on cellular surface which is able to combine adjacent cells, and the intracellular region which is able to link α- and β-catenin to the actin cytoskeleton sustaining cell shape and polarity, is regarded as an important EMT feature which plays critical roles in EMT by changing the components of intercellular adhesion and regulating diverse signaling pathways.[91]
E-cadherin downregulation and initiation and execution of EMT are caused by several microRNAs (miRNAs)[92] and various key EMT-inducing TFs including Snail1 (Snail), Snail2 (Slug), Twist, Zeb1, Zeb2 (Sip1), forkhead box C2, E47, Krüppel-like factor (KLF)4, and KLF8, goosecoid, Sox9, which interact with each other, and which are downstream in important signaling pathways activated by transforming GF-β (TGF-β), Wnt, Notch, integrin, interleukin (IL)-5, IL-6, FGF, STAT-3, EGF, hepatic GF (HGF), and NF-κB.[93,94] EMT is induced in epithelia by diverse EC stimuli including TGF-β, HGF, TNF-α, hypoxia inducible factor 1-α, and inflammatory signals from surrounding microenvironment, which activate GF and chemokine receptors, the downstream signaling cascades, and several TFs.[95] Transduction of exogenous WNT5A into GC cells upregulates EMT-related genes, indicating that WNT5A regulates GC EMT.[96] Downregulation of Wnt5a by EGF, which increases Arf6 and ERK activity, is necessary for EGF-induced EMT in GC cells.[97] The nuclear expression and transcriptional activity of Snail and β-catenin are increased by inhibitory phosphorylation of glycogen synthase kinase-3β (GSK-3β) at Ser9 by FasL-induced EC signal-regulated kinase (ERK)/MAPK signaling. Snail associates with β-catenin in the nucleus and thus increases β-catenin transcriptional activity.[98] Amplification of ERBB2, MET, FGFR2, PIK3CA, AKT1, WNT2, WNT2B, and WNT8B, and downregulation of SFRP1 and PAR3/PAR6/a PKC complex could lead to GC EMT also through GSK3β inhibition.[99] Aquaporin 3 (AQP3) upregulation represses E-cadherin, thus promoting EMT in human GC. It also upregulates the expression of vimentin and fibronectin in vitro. The PI3K/AKT/SNAIL signaling pathway is likely involved in the EMT induction by AQP3 in GC.[100 ] Caveolin-1 is modulated by HSP90 and functions as a crucial regulator of GC EMT.[101] Insulin-like GF I (IGF-I) induces EMT by upregulating the levels of Zeb2, which is dependent on the PI3K/Akt signaling pathway in GC cells. Positive expression of CD146 is strongly associated with loss of E-cadherin and acquisition of the mesenchymal markers nuclear β-catenin and vimentin, suggesting that CD146 might promote EMT.[102]
GC is one of the typical oxidative stress-related malignancies.[103] Hypoxia is also a significant GC EMT inducer. Under hypoxia, E-cadherin decreases and N-cadherin, vimentin, Snail, Sox2, Oct4, and Bmi1 increase, indicating that the hypoxic microenvironment induces EMT, accompanied by cytoskeleton remodeling.[104] Recent evidence indicates that EMT is a key GC progression driver, and plays a fundamental role during early steps of GC invasion, metastasis, and relapse. EMT-induced cell migration and reach their metastatic niche through both the lymphatic system and blood.[105] Histone demethylase Jumonji domain containing protein 2B (JMJD2B) promotes EMT and GC invasion and metastasis throughβ-catenin-induced H3K9 demethylation, which causes vimentin upregulation, implicating JMJD2B as a potential target for reversing EMT and intervening GC progression.[106]
LOH (Loss of Heterozygosity)
This describes a genetic phenomenon often seen with tumor suppressor genes in cancer. Since the human karyotype is diploid, mutation of one allele of a tumor suppressor gene is not sufficient to cause cancer. In heterozygous individuals, the wildtype allele will provide for a functional phenotype. However, when a“second hit” occurs, for example, through mis-segregation of chromosomes, this individual (or cell) may lose its “heterozygosity,” leading to a full cancerous phenotype. Recently, Karaman et al.[107] found significant correlation between prevalence of 17p (TP53) LOH in gastric precancerous lesions, indicating that loss of TP53 could be an early event in gastric carcinogenesis.[107]
Research in recent years demonstrated that although PTEN mutations in GC are rare, LOH is more frequent. Byun et al. (2003) found decreased expression of PTEN and upto 47% LOH in 33% (5/15) GC cell lines and 36% (22/55) GC tissue samples.[108] LOH rate was significantly higher in advanced than in early GC (63–18%); it was also significantly higher in poorly differentiated than highly and moderately differentiated GC (69–29%). This suggests that complete functional inactivation of PTEN is not necessary to cause gastric carcinogenesis, loss of one allele is sufficient.[108] LOH occurs in different stages of GC, genetic mutations could be detected only in advanced GC. Both gene mutations and LOH can promote tumor invasion and metastasis. Some studies have shown that the LOH status of PTEN can impact sensitivity to chemotherapy and hence, the prognosis in patients with GC. It may also be used as an independent prognostic tool. There are several putative TSGs, the hypermethylated in cancer 1 (HIC1) gene and TOB1 gene. The HIC1 gene is an interesting candidate TSG located in the overlapping LOH subregion of LOH in chromosome 17p13.3. It encodes a zinc-finger transcription regulator that contains an NH2-terminal BTB-POZ domain characteristic of a family of repressors. The gene is ubiquitously expressed in normal tissues, but a decrease or loss of expression was found in several types of tumors excluding GC.[109] Indeed, there is accumulating evidence indicating that a change in HIC1 expression through epigenetic mechanisms has an important role in tumor progression. The patterns of monoallelic methylation associated with a partial loss of HIC1 expression and biallelic methylation, together with a marked loss in HIC1 expression, were seen in these GCs. The concomitant loss or decreased expression and promoter methylation of HIC1 indicated that HIC1 may be silenced by aberrant DNA methylation, and could be a target for inactivation by epigenetic events in gastric tumorigenesis.[110] Another notable candidate gene in the overlapping LOH subregion in chromosome 17q21.33 (SR3) is TOB1 (transducer of ERBB-2, 1). TOB1 is a member of the TOB/BTG antiproliferation protein family. Overexpression of the TOB family proteins results in cell growth retardation,[109] while TOB LOH and decreased TOB expression were observed in lung cancer tissues.[110]
Genetic analyses of LOH helped to identify the chromosomal location of many tumor suppressor genes. LOH analyses have identified several arms and regions of chromosomes that contain or potentially harbor tumor suppressor genes important in gastric tumorigenesis. Chromosome 3p was the most frequently lost locus in LOH analysis of over 100 archived stomach cancers.[111] Moreover, three distinct regions of chromosome 4q were found to be frequently lost in gastroesophageal junctional adenocarcinomas.[111] LOH is also a marker of chromosomal instability and might indicate a second inactivation hit of cancer suppressor genes. Several LOH studies demonstrated that the extent of chromosomal loss appeared to be of prognostic significance. LOH has been shown to relate to cancer progression, where a transition from LOH-L to LOH-H is thought to reflect an increase in chromosomal instability during tumor advancement.
The highest LOH frequencies have been identified at 1p, 2q, 3p, 4p, 5q, 6p, 7p, 7q, 8p, 9p, 11q, 12q, 13q, 14q, 17p, 18q, 21q, and 22q chromosome regions in gastric carcinomas.[111] The main consequence of LOH is loss of genes, such as tumor suppressors, cell cycle regulators, DNA repair genes, and other genes implicated in the maintenance of cell cycle, and/or integrity of DNA. LOH studies have revealed that allelic imbalance of chromosome 17 loci occurs frequently in sporadic GC. Most of the LOH studies performed on chromosome 17 to date have used only a limited number of markers, and a detailed deletion map of overlapping regions on chromosome 17 has not yet been made in sporadic GC.
Microsatellite instability
Another type of genomic instability, commonly recognized in GC, is MSI. MSI is characteristic for hereditary type of GC, developed in the context of a smaller subset of sporadic cancers ranging from 25% to 50%.[112] Patients with MSI phenotype exhibit a high frequency of replication errors resulting in insertions/deletions of nucleotides within microsatellite repeats in tumor tissues.[112] In GCs, MSI is mostly caused by the epigenetic alterations in the mismatch repair (MMR) genes.[113,114] Consequently, the impaired MMR system fails to fulfill its task, resulting in multiple mutations within cell growth regulating genes (TGF-β RII, IGFIIR, RIZ, TCF4, and DP2), apoptosis genes (BAX, BCL10, FAS, CASPASE5, and APAF1), and DNA repair genes (hMSH6, hMSH3,MED1, RAD50, BLM, ATR, and MRE11). The high incidence of MSI in GCs (MSI-H GC) is more likely to occur at an antral location, in the intestinal type, in the expanding type, and with H. pylori seropositivity, and correlates with a lower prevalence of lymph-node metastases.[115-117] These errors are detected and repaired by a complex of MMR proteins. Inactivation or deficiency of one or more MMR genes, particularly MLH1 or MSH2, induces development of MSI phenotype, which often leads to additional genetic changes, namely, inactivation of tumor suppressor genes and LOH.[118,119]
Impairment of MMR can occur (1) by mutational inactivation of one or two MMR genes or (2) by epigenetic inactivation of MMR genes (CIMP). Genomes of GCs exhibiting MSI are characterized by the presence of multiple frameshift mutations in many genes at variable frequencies.[120] Genes that were frequently found to be altered as a consequence of impaired MMR are implicated in cell cycle regulation and apoptosis (TGF-β RII, IGFIIR, TCF4, RIZ, BAX, CASPASE5, FAS, BCL10, and APAF1) or are involved in genomic integrity maintenance (MSH6, MSH3, MED1, RAD50, BLM, ATR, and MRE11).[119,121] The alterations in these genes further promote genetic instability and enhance the development of malignant phenotype. MSI seems to be a promising tool to identify patients with genetic instability and patients with precancerous lesions because it occurs in both gastric adenoma and intestinal metaplasia.[112]
Epigenetic changes
Broadly, epigenetics refer to alteration in gene expression which is not regulated by the changes in the DNA sequences. DNA methylation and histone modifications are commonly studied epigenetic events. In the present era, the term epigenetics has broadened to include heritable and transient/reversible changes in gene expression that is not accompanied by a change in the DNA sequence. Comprehensive understanding of different biological activity such asDNA methylation, chromatin structure, transcriptional activities, and histone modification has contributed in the development of epigenetics. Two major epigenetic modifications include DNA methylation and chromatin remodeling. While DNA methylation is a chemical change in the DNA sequence that most commonly occurs at cytosine moiety of CpG dinucleotides, chromatin remodeling occurs throughhistone modifications (primarily on the N- terminal tails) that ultimately affect the interaction of DNA with chromatin modifying protein. Both DNA methylation and histone modifications are associated with silencing critical TSGs and activating oncogenes involved in cancer.[122-124]
HYPERMETHYLATION
DNA methylation is a reversible chemical modification of the cytosine in the CpG islands of promoter sequences, catalyzed by a family of DNA methyltransferases. DNA methylation does not change the genetic information but it just alters the readability of the DNA and results in the inactivation of gene by subsequent transcript repression.[125] In general, CpG island methylation is associated with gene silencing. The methylated CpG Island also recruits histone deacetylases (HDAC) and other factors involved in transcriptional silencing.[122] Inactivation of TSGs through hypermethylation of CpG islands within promoter regions is a major event in carcinogenesis.[122] It includes various genes involved in different cellular process such as cell cycle regulation (p16NK4a, CDKN2b/p15INK4b, and p14ARF) which is hypermethylated in human cell lines and primary tumors, DNA repair genes (hMLH 1 and MGMT) were observed aberrantly methylated at their promoter regions, cell-cell/ cell matrix adhesion genes (E-cadherin, H-cadherin and adenomatous polyposis coli [APC]), apoptosis (death-associated protein kinase [DAPK], TMS1, and Caspase-8), and angiogenesis (THBS-1 and p73).[126] Silencing of p16INK4a by promoter hypermethylation has also been reported in gastric carcinoma. CDKN2A hypermethylation may contribute to the malignant transformation of gastric precursor lesions. Hypermethylation of DAPK was observed in intestinal, diffuse, and mixed type of GC and correlated with the presence of LN metastasis, advanced stage, and poor survival.[127] The epigenetic silencing of XAF1 gene by aberrant promoter methylation is reported in GC.[128] Caspase-1 (IL-1 beta-converting enzyme), a member of the cysteine protease family, shows loss of expression in 19.3% cases of gastric carcinoma[129] and the expression is reversed on 5-aza 2’deoxycytidine and/or trichostatin treatments in GC cell line. The expression of TSPYL5 mRNA was frequently downregulated due to hypermethylation of its CpG Island.[129] Recently, Sepulveda et al. (2016)[130] identified 13 genes (BRINP1, CDH11, CHFR, EPHA5, EPHA7, FGF2, FLI1, GALR1, HS3ST2, PDGFRA, SEZ6L, SGCE, and SNRPN) which are hypermethylated in gastric carcinoma as compared with normal mucosa, which in turn may be useful in developing diagnostic and prognostic tool for this lethal malignancy.
Hypomethylation of specific genes also contribute to gastric carcinogenesis. Global hypomethylation of the genome was initially thought to be an exclusive event in cancer development.[131] The loss of methylation in cancer is mainly due to hypomethylation of repetitive DNA sequences. During the development of neoplasm, the degree of hypomethylation of genomic DNA increases as the lesion progress from a benign disease to metastatic.[122,132] Three mechanisms of DNA hypomethylation have been proposed in the development of cancers. First, it increases the genomic instability, second by reactivation of transposable elements, and third by loss of imprinting. Demethylation of DNA can favour mitotic recombination, leading to deletions, translocations, and chromosomal instability.[122] Demethylation of MAGE, synuclein-γ (SNCG), and cyclin D2 has been described in gastric carcinoma.[133] SNCG demethylation is common in cases with LN metastasis.[130] Hypomethylation of cyclin D2 promoter was found in 71% cases of gastric carcinoma. This event is more common in Stage-III and IV tumor than in Stage-I and II tumor.[134]
In parallel to global hypomethylation, hypermethylation of CpG Island also has silencing effect on miRNAs. MicroRNAs are short, 18–22 nucleotide, noncoding RNAs that regulate many cellular functions including cell proliferation, apoptosis, and differentiation by silencing specific target genes through translational repression or mRNA degradation.[127,126]
HISTONE MODIFICATION
In normal cell, a precise balance maintains nucleosomal DNA in either an active/acetylated or an inactive/deacetylated form. This adequate balance is controlled by acetylating enzymes (histone acetyltransferases) and deacetylating enzymes (HDACs). The other modification includes methylation of arginine and lysine residues of histones. This methylation is catalyzed by histone methyltransferase and the process is involved in the regulation of a wide range of gene activities and chromatin structures. In general, lysine methylation at H3K9, H3K27, and H4K20 is associated with gene silencing whereas methylation at H3K4, H3K36, and H3K79 isassociated with gene activation.[124]
Increasing evidence suggests that epigenetic changes play a key role in cancer development including GC. CpG island methylation phenotype as high CIMP (CIMP-H) play an important role in gastric carcinoma progression.
HEREDITARY GC
While the great majority of GCs is sporadic, familial aggregation occurs in about 10%of the cases and out of these only 1–3% clearly constitutes hereditary form. Hereditary GC includes syndromes such as hereditary diffuse GC, gastric adenocarcinoma and proximal polyposis of the stomach (GAPPS), and familial intestinal GC (FIGC). GC has also been identified as part of other hereditary cancer syndromes such as hereditary non-polyposis colorectal cancer, Li-Fraumeni syndrome, familial adenomatous polyposis, and Peutz-jeghers syndrome.[135]
HDGC is one of the best genetically characterized forms of hereditary GC. Heterozygous germline CDH1 (E-cadherin) mutations, including frameshifts, splice site, nonsense, and missense mutations as well as large rearrangements, were until recently the only unknown causative alterations of HDGC representing upto 40% of patients belonging to families that fulfill the clinical criteria for HDGC.[136] Germline variants of genes also cause GCs.[137] Hereditary diffuse GC is a well-known familial GC which is linked to CDH1 (E-cadherin) gene variants.[137] The physical position of the germline mutation of CDH1 gene vary according to ethnic and geographical differences.[137] There are 100 variants of the CTNNA1 (α-catenin), a CDH1 binding protein also cause HDGC.[138]
HDGC is an autosomal dominant cancer susceptibility syndrome characterized by DGC which is principally caused by inactivating germline mutations in CDH1 gene.[139] CDH1 gene encodes E-cadherin has major function in cell-cell adhesion, maintenance of epithelial architecture, cell polarity, and regulation of intracellular signaling pathways. The pathogenic mutation in CDH1 shows 70% risk of developing DGC by age of 80 years by birth.[140] Histopathology of advanced HDGC is comparable to sporadic DGC, though the presence of typical precursor lesions, insitu or pagetoid signet-ring cells, isspecific for CDH1- mutation related HDGC. Early HDGC shows indolent (showing no real interest/efforts) phenotype, while advanced HDGC display an aggressive phenotype with a mixture of pleomorphic cells, increased proliferation and aberrant P53 expression.[141] There are 10–40% of families who fulfills current genetic testing criteria have CDH1 germline mutations.[114] Although it is not known exactly how many HDGC families with germline CDH1 mutations have been diagnosed worldwide to date, over 155 different CDH1 germline mutations have been reported in HDGC families.[142] These mutations span the length of CDH1 and no major hotspots have been identified, although some including c.1137G>A and c.792C>T have been observed in several unrelated families.[140,142]
CDH1 mutation positive patients who postponed a prophylactic gastrectomy, but underwent regular surveillance endoscopy, had a ten-fold increased risk of DGC compared with individuals at risk of HDGC without a mutation.[143] CDH1 negative families, fulfilling HDGC criteria, often present monoallelic expression of CDH1 in the germline, which mimics functionally the presence of a CDH1 truncating mutation.[145]The underlying genetic mechanism causing this expression imbalance is still elusive, this finding hints toward CDH1 involvement in a larger fraction of HDGC families. The complete inactivation of the CDH1 gene required for tumor initiation occurs mainly through promoter methylation in primary cancer and loss-of-heterozygosity in lymph node metastases.[145,146] The ongoing search for novel HDGC predisposing germline aberrations identifies new variants in candidate genes using multiplexed panel and whole exome sequencing.[140,147-149] Germline CTNNA1 mutations have been found in families with HDGC.[149,150] CTNNA1, encoding α-catenin, is involved in cell adhesion, and forms a complex with β-catenin to bind the cytoplasmic domain of E-cadherin to the cytoskeleton.[150] New hereditary GC genes are the most promising genes which seems to be the DNA double-strand break repair genes PALB2, BRCA2, RAD51C, and ATM,[140,147-151] which earlier reported in breast cancer families. PALB2 and RAD51C are critical in homologous recombination which is a major DNA repair pathway. While designing gene panels to identify HDGC, these new candidate genes can be considered to broaden the understanding of genetic causes of this disease.
Syndromes with fundic gland polyps and increased GC risk include GAPPS, an autosomal-dominant heritable form of GC, which is caused by point mutations in the APC gene promoter 1B.[152,153] Affected patients shows specific clinicopathologic phenotype of fundic gland with about 10–100s of polyps involving oxyntic mucosa, occasional hyperplastic, and adenomatous polyps, with an increased risk for intestinal-type (WHO tubular type) or mixed-type gastric adenocarcinoma.[152] The gastric antrum and pylorus are typically spared. In this syndrome other histological features involves hyperproliferative aberrant pits, characterized by disorganized proliferations of oxyntic glands high up in the mucosa with an increased proliferative activity.[154] The dysplasia in GAPPS is of gastric foveolar phenotype with positive staining for MUC5AC and MUC6.[154]
FIGC intestinal-type gastric carcinoma patients have also shown familiar clustering. The diagnosis in young patients is strongly associated with a family history of GC in first degree relatives.[155] Familial aggregation of H. pylori infection seems to be related to incidence of intestinal-type GC.[155] The diagnosis should be considered when there is a history of intestinal-type GC in families without polyposis. A single family with clustering of intestinal-type GC and heterozygous, mutations in the immunity gene IL12RB1 has been reported, but further research is required to determine whether such mutations increase GC risk.[156]
FIGC follows an autosomal dominant inheritance pattern[158] and diagnostic criteria[146] are stratified on the basis of the population GC incidence. In low-incidence countries, diagnosis requires that a patient has at least two first-degree or second-degree relatives with intestinal GC, one by 50 years of age, or has three or more first-degree or second-degree relatives diagnosed with GC at any age.[158,159] In high-incidence countries, diagnosis requires three criteria: (1) At least three relatives with intestinal GCs with one of them being a first-degree relative of the other two, (2) another criteria are occurrence of GC in at least two generations, and (3) and diagnosis of GC before 50 years of age in at least one patient.[95,159]
CONCLUSION
GC is a collection of different molecular entities and its landscape is enormously complex, but recent efforts and knowledge enabled for the development of more modern and solid therapeutic approaches. Several important advances have been achieved in GC genetics. The improvement in our understanding of the molecular genetics of GC has greatly hastened over the last decades, enabling us to redefine the disease at the molecular level. These findings may lead to the identification of high-risk groups that can be targeted for early interventions which may improve our understanding of tumorigenesis and will ultimately lead to improved outcomes for this common malignancy. Molecular classifications, especially TGCA and ACRG, opened the doors wide on the complete comprehension of the complex genetic landscape of GC. Recent genomic and epigenomic profiling studies provides an improved molecular understanding of GC. In the above article, the characterization and classification of GC at molecular and genetic level would further support that this disease is highly heterogeneous. Researchers and clinicians should take advantage of the information provided by these studies to both design and test potential screening markers and new targeted therapeutic approaches.
Declaration of patient consent
Patient’s consent not required as there are no patients in this study.
Financial support and sponsorship
Nil.
Conflicts of interest
There are no conflicts of interest.
References
- Cancer incidence and mortality worldwide: Sources, methods and major patterns in GLOBOCAN 2012. Int J Cancer. 2015;136:E359-86.
- [CrossRef] [PubMed] [Google Scholar]
- Prospective study of educational background and stomach cancer in Japan. Prev Med. 2010;35:121-7.
- [CrossRef] [PubMed] [Google Scholar]
- Dietary factors and gastric cancer risk: Hospital-based case control study. J BUON. 2010;15:89-93.
- [Google Scholar]
- Carcinoma of the stomach: A review of epidemiology, pathogenesis, molecular genetics and chemoprevention. World J Gastrointest Oncol. 2012;4:156-69.
- [CrossRef] [PubMed] [Google Scholar]
- Gastric cancer: Preoperative TNM staging with individually adjusted computed tomography scanning phase. J Comput Assist Tomogr2016;. ;40:160-6.
- [CrossRef] [PubMed] [Google Scholar]
- NCCN Clinical Practice Guidelines in Oncology (NCCN Guidelines®) Gastric Cancer. 2015. Available from: http://www.nccn.org [Last accessed on 17 Jan 2019]
- [Google Scholar]
- Molecular classification of gastric cancer: A new paradigm. Clin Cancer Res. 2011;17:2693-701.
- [CrossRef] [PubMed] [Google Scholar]
- Gastric cancer: A primer on the epidemiology and biology of the disease and an overview of the medical management of advanced disease. J Natl Compr Canc Netw. 2010;8:437-47.
- [CrossRef] [PubMed] [Google Scholar]
- Patholomorphological diagnosis, Definition and gross classification of early gastric cancer. Gann Monogr Cancer Res. 1971;11:53-5.
- [Google Scholar]
- The Paris endoscopic classification of superficial neoplastic lesions: Esophagus stomach and colon: November 30 to December 1, 2002. Gastrointest Endosc. 2003;58:S3-43.
- [CrossRef] [Google Scholar]
- Characteristics of gastric cancer invading to the proper muscle layer--with special reference to mortality and cause of death. Jpn J Clin Oncol. 1985;15:499-503.
- [Google Scholar]
- The two histological main types of gastric carcinoma: Diffuse and so-called intestinal-type carcinoma an attempt at a histo-clinical classification. Acta Pathol Microbiol Scand. 1965;64:31-49.
- [CrossRef] [PubMed] [Google Scholar]
- Current perspectives on gastric cancer. Gastroenterol Clin North Am. 2016;45:413-28.
- [CrossRef] [PubMed] [Google Scholar]
- The Cancer Genome Atlas Research Network, Comprehensive molecular characterization of gastric adenocarcinoma. Nature. 2014;513:202-9.
- [CrossRef] [PubMed] [Google Scholar]
- Molecular analysis of gastric cancer identifies subtypes associated with distinct clinical outcomes. Nat Med. 2015;21:449-56.
- [CrossRef] [PubMed] [Google Scholar]
- Distinct subtypes of gastric cancer defined by molecular characterization include novel mutational signatures with prognostic capability. Cancer Res. 2016;76:1724-32.
- [CrossRef] [PubMed] [Google Scholar]
- EBV infection in cardiac and non-cardiac gastric adenocarcinomas is associated with promoter methylation of p16, p14 and APC, but not hMLH1. Anal Cell Pathol (Amst). 2010;33:143-9.
- [CrossRef] [Google Scholar]
- A comprehensive survey of genomic alterations in gastric cancer reveals systematic patterns of molecular exclusivity and co-occurrence among distinct therapeutic targets. Gut. 2012;61:673-84.
- [CrossRef] [PubMed] [Google Scholar]
- Molecular heterogeneity and receptor coamplification drive resistance to targeted therapy in MET amplified esophagogastric cancer. Cancer Discov. 2015;5:1271-81.
- [CrossRef] [PubMed] [Google Scholar]
- Comprehensive analyses using next-generation sequencing and immunohistochemistry enable precise treatment in advanced gastric cancer. Ann Oncol. 2016;27:127-33.
- [CrossRef] [PubMed] [Google Scholar]
- Expression profiles of HER2, EGFR, MET and FGFR2 in a large cohort of patients with gastric adenocarcinoma. Gastric Cancer. 2015;18:227-38.
- [CrossRef] [PubMed] [Google Scholar]
- The nationwide cancer genome screening project in Japan SCRUM-Japan GI-SCREEN: Efficient identification of cancer genome alterations in advanced gastric cancer (GC) J Clin Oncol. 2018;36:4050.
- [CrossRef] [Google Scholar]
- Retrospective review using targeted deep sequencing reveals mutational differences between gastroesophageal junction and gastric carcinomas. BMC Cancer. 2015;15:1.
- [CrossRef] [PubMed] [Google Scholar]
- Recent progress in the study of methylated tumor suppressor genes in gastric cancer. Chin J Cancer. 2013;32:31.
- [CrossRef] [PubMed] [Google Scholar]
- CpG island hypermethylation in progression of esophageal and gastric cancer. Cancer. 2006;106:483-93.
- [CrossRef] [PubMed] [Google Scholar]
- Alterations of tumor suppressor and tumor-related genes in the development and progression of gastric cancer. World J Gastroenterol. 2006;12:192.
- [CrossRef] [PubMed] [Google Scholar]
- Epigenetics of gastric cancer In: Verma M, ed. Cancer Epigenetics Risk Assessment, Diagnosis, Treatment, and Prognosis. New York: Springer; 2015. p. :783-99.
- [Google Scholar]
- Exom sequencing identifies frequent mutation of ARID1A in molecular subtypes of gastric cancer. Nat Genet. 2011;43:1219-23.
- [CrossRef] [PubMed] [Google Scholar]
- Whole-genome sequencing and comprehensive molecular profiling identify new driver mutations in gastric cancer. Nat Genet. 2014;46:573-82.
- [CrossRef] [PubMed] [Google Scholar]
- Evolutionary recombination hotspot around GSDMLGSDM locus is closely linked to the oncogenomic recombination hotspot around the PPP1R1.BERBB2-GRB7 amplicon. Int J Oncol. 2004;24:757-63.
- [CrossRef] [Google Scholar]
- Intragenic mutations of CDKN2B and CDKN2A in primary human esophageal cancers. Hum Mol Genet. 1995;4:1883-7.
- [CrossRef] [PubMed] [Google Scholar]
- Mutagenic and epigenetic effects of DNA methylation. Mutat Res. 1997;386:107-18.
- [CrossRef] [Google Scholar]
- Progress of research on tumour suppressor genes. Hokkaido Igaku Zasshi. 1996;71:137.
- [Google Scholar]
- Tumour suppressor gene expression correlates with gastric cancer prognosis. J Pathol. 2003;200:39-46.
- [CrossRef] [PubMed] [Google Scholar]
- Genomania of p53 protein in gastric cancer. J Clin Gastroenterol. 1996;22:170-3.
- [CrossRef] [PubMed] [Google Scholar]
- p53 mutations in the nonneoplastic mucosa of the human stomach showing intestinal metaplasia. Int J Cancer. 1996;69:28-33.
- [CrossRef] [Google Scholar]
- Oncogenic and therapeutic targeting of PTEN loss in bone malignancies. J Cell Biochem. 2015;116:1837-47.
- [CrossRef] [PubMed] [Google Scholar]
- Roles of PTEN (phosphatase and tensin homolog) in gastric cancer development and progression. Asian Pac J Cancer Prev. 2014;15:17-24.
- [CrossRef] [PubMed] [Google Scholar]
- Mutation analysis of tumor suppressor gene PTEN in patients with gastric carcinomas and its impact on PI3K/AKT pathway. Oncol Rep. 2010;24:89-95.
- [CrossRef] [Google Scholar]
- E-cadherin dysfunction in gastric cancer cellular consequences, clinical applications and open questions. FEBS Lett. 2012;586:2981-9.
- [CrossRef] [PubMed] [Google Scholar]
- The clinicopathological significance of CDH1 in gastric cancer: A meta-analysis and systematic review. Drug Des Devel Ther. 2015;9:2149-57.
- [CrossRef] [PubMed] [Google Scholar]
- Pathology of gastric cancer and its precursor lesions. Gastroenterol Clin North Am. 2013;42:261-84.
- [CrossRef] [PubMed] [Google Scholar]
- Somatic mutations and deletions of the E-cadherin gene predict poor survival of patients with gastric cancer. J Clin Oncol. 2013;31:868-75.
- [CrossRef] [PubMed] [Google Scholar]
- Association between the expression of T-cadherin and vascular endothelial growth factor and the prognosis of patients with gastric cancer. Mol Med Rep. 2015;12:2075-81.
- [CrossRef] [PubMed] [Google Scholar]
- MicorRNA 106b~25 clus ter and gastric cancer. Surg Oncol. 2013;22:e7-10.
- [CrossRef] [PubMed] [Google Scholar]
- Fbxw7 tumor suppressor: A vital regulator contributes to human tumorigenesis. Medicine (Baltimore). 2016;95:e2496.
- [CrossRef] [PubMed] [Google Scholar]
- Mutational analysis of the hCDC4 gene in gastric carcinomas. Eur J Cancer. 2006;42:2369-73.
- [CrossRef] [PubMed] [Google Scholar]
- p53-Altered FBXW7 expression determines poor prognosis in gastric cancer cases. Cancer Res. 2009;69:3788-94.
- [CrossRef] [PubMed] [Google Scholar]
- Fbxw7 regulates tumor apoptosis, growth arrest and the epithelial-tomesenchymal transition in part through the RhoA signaling pathway in gastric cancer. Cancer Lett. 2016;370:39-55.
- [CrossRef] [PubMed] [Google Scholar]
- The human c-myc oncogene-structural consequences of translocation into the IgH locus in Burkitts lymphoma. Cell. 1983;34:779-87.
- [CrossRef] [Google Scholar]
- The c-Myc target gene network. Semin Cancer Biol. 2006;4:253-64.
- [CrossRef] [PubMed] [Google Scholar]
- MYC and gastric adenocarcinoma carcinogenesis. World J Gastroenterol. 2008;14:5962-8.
- [CrossRef] [PubMed] [Google Scholar]
- Impact of excision repair cross-complementing gene 1 (ERCC1) on the outcomes of patients with advanced gastric cancer: Correlative study in Japan clinical oncology group trial JCOG9912. Ann Oncol. 2013;10:2560-5.
- [CrossRef] [PubMed] [Google Scholar]
- Recent patterns in gastric cancer: A global overview. Int J Cancer. 2009;125:666-73.
- [CrossRef] [PubMed] [Google Scholar]
- Ramucirumab monotherapy for previously treated advanced gastric or gastro-oesophageal junction adenocarcinoma (REGARD): An international, randomised, multicentre, placebo-controlled, phase 3 trial. Lancet. 2014;383:31-9.
- [CrossRef] [Google Scholar]
- The potential role of Bcl-2 expression, apoptosis and cell proliferation (Ki-67 expression) in cases of gastric carcinoma and correlation with classic prognostic factors and patient outcome. Anticancer Res. 2009;29:703-9.
- [Google Scholar]
- PRR11 is a novel gene implicated in cell cycle progression and lung cancer. Int J Biochem Cell Biol. 2013;45:645-56.
- [CrossRef] [PubMed] [Google Scholar]
- PRR11 is a prognostic marker and potential oncogene in patients with gastric cancer. PLoS One. 2015;10:e0128943.
- [CrossRef] [PubMed] [Google Scholar]
- The prognostic significance of altered cyclin-dependent kinase inhibitors in human cancer. Annu Rev Med. 1999;50:401-23.
- [CrossRef] [PubMed] [Google Scholar]
- Abnormal expression of p16(INK4a), cyclin D1, cyclin-dependent kinase 4 and retinoblastoma protein in gastric carcinomas. J Surg Oncol. 2008;98:60-6.
- [CrossRef] [PubMed] [Google Scholar]
- Expression of cell-cycle regulatory proteins cyclin D1, cyclin E, and their inhibitorp21 WAF1/CIP1 in gastric cancer. J Pathol. 1999;189:186-93.
- [CrossRef] [Google Scholar]
- Prognostic implications of cyclin B1, p34cdc2, p27 (Kip1) and p53 expression in gastric cancer. Yonsei Med J. 2007;48:694-700.
- [CrossRef] [PubMed] [Google Scholar]
- Immunohistochemical analysis of pRb2/p130, VEGF, EZH2, p53, p16(INK4A), p27(KIP1), p21(WAF1), Ki-67 expression patterns in gastric cancer. J Cell Physiol. 2007;210:183-91.
- [CrossRef] [PubMed] [Google Scholar]
- Reduced expression of cyclin-dependent kinase inhibitor p27 Kip1 is associated with advanced stage and invasiveness of gastric carcinomas. Jpn J Cancer Res. 1997;88:625-9.
- [CrossRef] [PubMed] [Google Scholar]
- Apoptosis: A basic biological phenomenon with wide-ranging implications in tissue kinetics. Br J Cancer. 1972;26:239-57.
- [CrossRef] [PubMed] [Google Scholar]
- Cell death: The significance of apoptosis. Int Rev Cytol. 1980;68:251-306.
- [CrossRef] [Google Scholar]
- Apoptosisits significance in cancer and cancer therapy. Cancer. 1994;73:2013-26.
- [CrossRef] [Google Scholar]
- Apoptosis in the pathogenesis and treatment of disease. Science. 1995;267:1456-62.
- [CrossRef] [PubMed] [Google Scholar]
- Cloning of the chromosome breakpoint of neoplastic B cells with the t (14;18) chromosome translocation. Science. 1984;226:1097-9.
- [CrossRef] [PubMed] [Google Scholar]
- Involvement of the bcl-2 gene in human follicular lymphoma. Science. 1985;228:1440-3.
- [CrossRef] [PubMed] [Google Scholar]
- BCL-2 family members and the mitochondria in apoptosis. Genes Dev. 1999;13:1899-911.
- [CrossRef] [PubMed] [Google Scholar]
- Gastrokine 1 induces senescence through p16/Rb pathway activation in gastric cancer cells. Gut. 2012;61:43-52.
- [CrossRef] [PubMed] [Google Scholar]
- Low-dose aspirin reduces the gene expression of gastrokine-1 in the antral mucosa of healthy subjects. Aliment Pharmacol Ther. 2008;28:782-8.
- [CrossRef] [PubMed] [Google Scholar]
- Gastrokine 1 is abundantly and specifically expressed in superficial gastric epithelium, down-regulated in gastric carcinoma, and shows high evolutionary conservation. J Pathol. 2004;203:789-97.
- [CrossRef] [PubMed] [Google Scholar]
- Proteomics characterization of gastrokine 1-induced growth inhibition of gastric cancer cells. Proteomics. 2011;11:3657-64.
- [CrossRef] [PubMed] [Google Scholar]
- Proteomic alteration in gastric adenocarcinomas from Japanese patients. Mol Cancer. 2006;5:75.
- [CrossRef] [PubMed] [Google Scholar]
- Detection of ß-catenin, gastrokine-2 and embryonic stem cell expressed ras in gastric cancers. Int J Clin Exp Pathol. 2010;3:782-91.
- [Google Scholar]
- Decreased expression of gastrokine 1 in gastric mucosa of gastric cancer patients. World J Gastroenterol. 2014;20:16702-6.
- [CrossRef] [PubMed] [Google Scholar]
- Helicobacter pylori infection and administration of nonsteroidal anti-inflammatory drugs down-regulate the expression of gastrokine-1 in gastric mucosa. Turk J Gastroenterol. 2012;23:212-9.
- [CrossRef] [PubMed] [Google Scholar]
- Molecular expression of gastrokine 1 in normal mucosa and in Helicobacter pylori-related preneoplastic and neoplastic gastric lesions. Cancer Biol Ther. 2008;7:1890-5.
- [CrossRef] [PubMed] [Google Scholar]
- Inactivation of the gastrokine 1 gene in gastric adenomas and carcinomas. J Pathol. 2011;223:618-25.
- [CrossRef] [PubMed] [Google Scholar]
- Synergistic tumour suppressor activity of E-cadherin and p53 in a conditional mouse model for metastatic diffuse-type gastric cancer. Gut. 2012;61:344-53.
- [CrossRef] [PubMed] [Google Scholar]
- Cadherin switch in tumor progression. Ann N Y Acad Sci. 2004;1014:155-63.
- [CrossRef] [PubMed] [Google Scholar]
- Genetic testing by cancer site: Stomach. Cancer J. 2012;18:355-63.
- [CrossRef] [PubMed] [Google Scholar]
- A prospective study of total gastrectomy for CDH1-positive hereditary diffuse gastric cancer. Ann Surg Oncol. 2011;18:2594-8.
- [CrossRef] [PubMed] [Google Scholar]
- Hereditary diffuse gastric cancer: Updated clinical guidelines with an emphasis on germline CDH1 mutation carriers. J Med Genet. 2015;52:361-74.
- [CrossRef] [PubMed] [Google Scholar]
- FOXQ1 regulates epithelial-mesenchymal transition in human cancers. Cancer Res. 2011;71:3076-86.
- [CrossRef] [PubMed] [Google Scholar]
- A mesenchymal to epithelial transition initiates and is required for the nuclear reprogramming of mouse fibroblasts. Cell Stem Cell. 2010;7:51-63.
- [CrossRef] [PubMed] [Google Scholar]
- Regulatory networks defining EMT during cancer initiation and progression. Nat Rev Cancer. 2013;13:97-110.
- [CrossRef] [PubMed] [Google Scholar]
- Hallmarks of cancer: The next generation. Cell2011;. ;144:646-74.
- [CrossRef] [PubMed] [Google Scholar]
- WNT5A is a key regulator of the epithelial mesenchymal transition and cancer stem cell properties in human gastric carcinoma cells. Pathobiology. 2013;80:235-44.
- [CrossRef] [PubMed] [Google Scholar]
- EGF-reduced Wnt5a transcription induces epithelial-mesenchymal transition via Arf6-ERK signaling in gastric cancer cells. Oncotarget. 2015;6:7244-61.
- [CrossRef] [PubMed] [Google Scholar]
- Fas signaling promotes motility and metastasis through epithelialmesenchymal transition in gastrointestinal cancer. Oncogene. 2013;32:1183-92.
- [CrossRef] [PubMed] [Google Scholar]
- Epithelial-mesenchymal transition in gastric cancer (review) Int J Oncol. 2005;27:1677-83.
- [Google Scholar]
- Aquaporin 3 promotes epithelial-mesenchymal transition in gastric cancer. J Exp Clin Cancer Res. 2014;33:38.
- [CrossRef] [PubMed] [Google Scholar]
- Caveolin-1 promotes gastric cancer progression by up-regulating epithelial to mesenchymal transition by crosstalk of signalling mechanisms under hypoxic condition. Eur J Cancer. 2014;50:204-15.
- [CrossRef] [PubMed] [Google Scholar]
- CD146 expression correlates with epithelial-mesenchymal transition markers and a poor prognosis in gastric cancer. Int J Mol Sci. 2012;13:6399-406.
- [CrossRef] [PubMed] [Google Scholar]
- Inflammation-associated cancer development in digestive organs: Mechanisms and roles for genetic and epigenetic modulation. Gastroenterology. 2012;143:550-63.
- [CrossRef] [PubMed] [Google Scholar]
- Hypoxic microenvironment induces EMT and upgrades stem-like properties of gastric cancer cells. Technol Cancer Res Treat. 2015;15:60-8.
- [CrossRef] [PubMed] [Google Scholar]
- miR-23a inhibits E-cadherin expression and is regulated by AP-1 and NFAT4 complex during fas-induced EMT in gastrointestinal cancer. Carcinogenesis. 2014;35:173-83.
- [CrossRef] [PubMed] [Google Scholar]
- JMJD2B promotes epithelial-mesenchymal transition by cooperating with betacatenin and enhances gastric cancer metastasis. Clin Cancer Res. 2013;19:6419-29.
- [CrossRef] [PubMed] [Google Scholar]
- Frequent monoallelic deletion of PTEN and its reciprocal associatioin with PIK3CA amplification in gastric carcinoma. Int J Cancer. 2003;104:318-27.
- [CrossRef] [PubMed] [Google Scholar]
- A serine/threonine kinase p90rsk1 phosphorylates the anti-proliferative protein tob. Gen Cells2001;. ;6:131-8.
- [CrossRef] [PubMed] [Google Scholar]
- Alteration of expression or phosphorylation status of tob, a novel tumor suppressor gene product is an early event in lung cancer. Cancer Lett. 2003;202:71-9.
- [CrossRef] [PubMed] [Google Scholar]
- Mapping of genetic deletions on the long arm of chromosome 4 in human esophageal adenocarcinomas. Am J Pathol. 1999;154:1329-34.
- [CrossRef] [Google Scholar]
- Patterns of genomic instability in gastric cancer: Clinical implications and perspectives. Ann Oncol. 2006;17(Suppl 7):97-102.
- [CrossRef] [PubMed] [Google Scholar]
- CDH1 germline mutations and the hereditary diffuse gastric and lobular breast cancer syndrome: A multicentre study. J Med Genet. 2013;50:486-48.
- [CrossRef] [PubMed] [Google Scholar]
- Germline CDH1 deletions in hereditary diffuse gastric cancer families. Hum Mol Gene. 2009;18:1545-55.
- [CrossRef] [PubMed] [Google Scholar]
- Microsatellite instability as a tool for the classification of gastric cancer. Trends Mol Med2001;. ;7:76-80.
- [CrossRef] [Google Scholar]
- Microsatellite instability-associated mutations associate preferentially with the intestinal type of primary gastric carcinomas in a high-risk population. Cancer Res. 1996;56:4662-5.
- [Google Scholar]
- Gastric cancers of the microsatellite mutator phenotype display characteristic genetic and clinical features. Gastroenterology. 1999;116:1348-57.
- [CrossRef] [Google Scholar]
- MRE11 expression is impaired in gastric cancer with microsatellite instability. Carcinogenesis. 2004;25:2337-43.
- [CrossRef] [PubMed] [Google Scholar]
- Genomic and genetic alterations influence the progression of gastric cancer. WorldJ Gastroenterol. 2011;17:290-9.
- [CrossRef] [PubMed] [Google Scholar]
- Gastric carcinomas with microsatellite instability: Clinical features and mutations to the TGF-ß Type II receptor, IGFII receptor, and BAX genes. J Pathol. 1999;187:428-32.
- [CrossRef] [Google Scholar]
- Epigenetics and human disease. Int J Biochem Cell Bio. 2009;41:136-46.
- [CrossRef] [PubMed] [Google Scholar]
- Cytogenetic and molecular aspects of gastric cancer: Clinical implications. Cancer Lett. 2008;266:99-115.
- [CrossRef] [PubMed] [Google Scholar]
- Epigenetic biomarkers for human cancer: The time is now. Crit Rev Oncol Hematol. 2008;68:1-11.
- [CrossRef] [PubMed] [Google Scholar]
- Role of epigenetic alterations in cholangiocarcinoma. J Hepatobiliary Pancreat Surg. 2006;13:274-9.
- [CrossRef] [PubMed] [Google Scholar]
- Epigenetic alterations in head and neck cancer: Prevalence, clinical significance, and implications. Curr Oncol Rep. 2004;6:152-61.
- [CrossRef] [PubMed] [Google Scholar]
- Promoter hypermethylation of death associated protein-kinase gene associated with advance stage gastric cancer. Oncol Rep. 2005;13:937-41.
- [CrossRef] [PubMed] [Google Scholar]
- Hypermethylation of XIAP associated factor 1, a putative tumor suppressor gene from the 17p13.2 locus, in human gastric adenocarcinomas. Cancer Res. 2003;63:7068-75.
- [Google Scholar]
- Loss of caspase-1 gene expression in human gastric carcinomas and cell lines. Int J Oncol. 2005;26:1265-71.
- [CrossRef] [PubMed] [Google Scholar]
- High-definition CpG methylation of novel genes in gastric carcinogenesis identified by nextgeneration sequencing. Mod Pathol. 2016;29:182-93.
- [CrossRef] [PubMed] [Google Scholar]
- Micro-RNAs as oncogenes and tumour suppressors. N Engl J Med. 2005;353:1768-71.
- [CrossRef] [PubMed] [Google Scholar]
- DNA methylation: A marker for carcinogen exposure and cancer risk. Environ Health Prev Med. 2008;13:8-15.
- [CrossRef] [PubMed] [Google Scholar]
- Methylation status of ANAPC1, CDKN2A and TP53 promoter genes in individuals with gastric cancer. Braz J Med Biol Res. 2008;41:539-43.
- [CrossRef] [PubMed] [Google Scholar]
- Micro-RNA signatures in human cancers. Nat Rev Cancer. 2006;6:857-66.
- [CrossRef] [PubMed] [Google Scholar]
- Familial gastric cancer: Genetic susceptibility, pathology, and implications for management. Lancet Oncol. 2015;16:e60-70.
- [CrossRef] [Google Scholar]
- Hereditary diffuse gastric cancer: Updated clinical guidelines with an emphasis on germline CDH1 mutation carriers. J Med Genet. 2015;52:361-74.
- [Google Scholar]
- Genetic screening for familial gastric cancer. Hered Cancer Clin Pract. 2004;2:51-64.
- [CrossRef] [PubMed] [Google Scholar]
- An alpha-e-catenin (CTNNA1)mutation in hereditary diffuse gastric cancer. J Pathol. 2013;229:621-9.
- [CrossRef] [PubMed] [Google Scholar]
- E-cadherin germline mutations in familial gastric cancer. Nature. 1998;392:402-5.
- [CrossRef] [PubMed] [Google Scholar]
- Hereditary diffuse gastric cancer syndrome: CDH1 mutations and beyond. JAMA Oncol. 2015;1:23-32.
- [Google Scholar]
- Histopathological, molecular, and genetic profile of hereditary diffuse gastric cancer: Current knowledge and challenges for the future. Adv Exp Med Biol. 2016;908:371-91.
- [CrossRef] [PubMed] [Google Scholar]
- Hereditary diffuse gastric cancer in a Japanese family with a large deletion involving CDH1. Gastric Cancer. 2014;17:750-6.
- [CrossRef] [PubMed] [Google Scholar]
- A comparative study of endoscopic surveillance in hereditary diffuse gastric cancer according to CDH1 mutation status. Gastrointest Endosc. 2018;87:408-18.
- [CrossRef] [PubMed] [Google Scholar]
- Allele-specific CDH1 downregulation and hereditary diffuse gastric cancer. Hum Mol Genet. 2010;19:943-52.
- [CrossRef] [PubMed] [Google Scholar]
- Methylation of the CDH1 promoter as the second genetic hit in hereditary diffuse gastric cancer. Nat Genet. 2000;26:16-7.
- [CrossRef] [PubMed] [Google Scholar]
- Quantification of epigenetic and genetic 2nd hits in CDH1 during hereditary diffuse gastric cancer syndrome progression. Gastroenterology. 2009;136:2137-48.
- [CrossRef] [PubMed] [Google Scholar]
- Germline mutations in PALB2, BRCA1, and RAD51C, which regulate DNA recombination repair, in patients with gastric cancer. Gastroenterology. 2017;152:983-6.
- [CrossRef] [PubMed] [Google Scholar]
- Unraveling genetic predisposition to familial or early onset gastric cancer using germline whole-exome sequencing. Eur J Hum Genet. 2017;25:1246-52.
- [CrossRef] [PubMed] [Google Scholar]
- Genetic gastric cancer susceptibility in the international clinical cancer genomics community research network. Cancer Gene. 2017;216-217:111-9.
- [CrossRef] [PubMed] [Google Scholar]
- Alpha-catenin can form asymmetric homodimeric complexes and/or heterodimeric complexes with beta-catenin. J Biol Chem. 1997;272:27301-6.
- [CrossRef] [PubMed] [Google Scholar]
- Germline pathogenic variants in PALB2 and other cancer-predisposing genes in families with hereditary diffuse gastric cancer without CDH1 mutation: A whole-exome sequencing study. Lancet Gastroenterol Hepatol. 2018;3:489-98.
- [CrossRef] [Google Scholar]
- Gastric adenocarcinoma and proximal polyposis of the stomach (GAPPS): A new autosomal dominant syndrome. Gut. 2012;61:774-9.
- [CrossRef] [PubMed] [Google Scholar]
- Point mutations in exon 1B of APC reveal gastric adenocarcinoma and proximal polyposis of the stomach as a familial adenomatous polyposis variant. Am J Hum Genet. 2016;98:830-42.
- [Google Scholar]
- Neoplastic lesions of gastric adenocarcinoma and proximal polyposis syndrome (GAPPS) are gastric phenotype. Am J Surg Pathol. 2018;42:1-8.
- [CrossRef] [PubMed] [Google Scholar]
- Gastric cancer and family history. Korean J Intern Med. 2016;31:1042-53.
- [CrossRef] [PubMed] [Google Scholar]
- Gastric cancer in three relatives of a patient with a biallelic IL12RB1 mutation. Fam Cancer. 2015;14:89-94.
- [CrossRef] [PubMed] [Google Scholar]
- Hereditary gastric cancer syndromes. Surg Oncol Clin N Am. 2015;24:765-77.
- [CrossRef] [PubMed] [Google Scholar]
- Familial gastric cancer: Overview and guidelines for management. J Med Genet. 1999;36:873-80.
- [Google Scholar]
- Intratumoral heterogeneity, its contribution to therapy resistance and methodological caveats to assessment. Front Oncol. 2014;4:142.
- [CrossRef] [PubMed] [Google Scholar]