Translate this page into:
Potential therapeutic molecular targets for better outcomes for patients with lung cancer
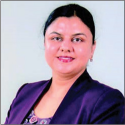
*Corresponding author: Dr. Punit Kaur, Associate Director, Precision Therapeutics Proteogenomics Diagnostics Center, Associate Professor, Department of Medicine, Division of Hematology/ Oncology, Eleanor N. Dana Cancer Center, University of Toledo College of Medicine and Life Sciences, Toledo, Ohio, United States. puneet9@gmail.com
-
Received: ,
Accepted: ,
How to cite this article: Mack ST, Morand SM, Ngo NT, Mitchell AC, McHugh MA, Kaur P, et al. Potential therapeutic molecular targets for better outcomes for patients with lung cancer. Int J Mol Immuno Oncol 2023;8:51-64.
Abstract
Lung cancer is the leading cause of death among all types of cancers in the U.S. and worldwide. Although more treatments have continuously been made over the past 30 years, the prognosis remains the same. This is mainly due to the late stage of the cancer at diagnosis and the eventual development of resistance seen in many types of cancer. The introduction of targeted therapies represents a major advancement in the treatment of tumor progression and an avenue to target its mechanisms of resistance. These agents are a novel therapeutic approach targeting specific molecules aberrantly expressed in these cells and through these mechanisms, alter the internal environment of the tumor cells. In this article, we present a critical discussion of the current clinical standards of treating non-small cell lung cancer (NSCLC), signal pathways of how these tumors acquire resistance, potential drug targets for these pathways, and the role heat shock proteins (HSP) play in NSCLC. Targeting HSP, phosphatidylinositol-3-kinase pathway anomalies, multidrug resistance protein 1 overexpression, and using these therapies to examine possible cooperative properties to resensitize tumor cells to chemotherapy or apoptosis are a worthwhile therapeutic direction. This is because some therapies may play a role as an adjuvant, while others may have a direct role in inducing the apoptotic effects. Therefore, combination therapeutics are an ideal direction to take in drug discovery. Comprehensive knowledge on current clinical standards of treating NSCLC, treatment resistance, novel drug targets, and the role HSP will allow for repurposing of current drugs on the market or develop completely new gene targets and therapies with more efficacy or a larger therapeutic index.
Keywords
5-Aza-CdR
Aminoxyrone
Copanlisib
Heat shock proteins
Lung cancer
KRAS
Molecular targets
MRP1 inhibitor
Norcantharidin
Pan-class I phosphoinositide 3-kinase inhibitor
Resistance
Reversan
INTRODUCTION
While there have been significant research and advancements in the treatment of lung cancer, it remains the highest mortality of any cancer in both men and women. As the third most common, behind breast, and prostate cancer, it is estimated that there will be 228,150 new cases in 2019. With an estimated 142,670 deaths in 2019, lung cancer’s death rates exceed that of all three of the other most common cancers combined, colon, breast, and prostate.[1] Overall, there are two main types of lung cancer, small cell lung carcinoma (SCLC), and non-small cell lung carcinoma (NSCLC). They account for 15% and 85% of all lung cancers, respectively. NSCLC is then further classified into three categories: Squamous cell carcinoma, adenocarcinoma, and large-cell carcinoma. Of NSCLCs, adenocarcinoma is the most common as it comprises about 40%. The next most common is squamous cell carcinoma at 30%, followed by large cell carcinoma at 15%.[2] The remaining percentage consists of many subtypes such as pleomorphic, carcinoid tumor, salivary gland carcinoma, and unclassified carcinoma, but they are all much less common. SCLC and NSCLC are very different diseases due to the fact they have different cell origins.
NSCLC cells originate from the typical lung parenchymal while SCLC is neuroendocrine cells with potential diverse functions. This cancer does not just have a large impact in the United States; it also shows similar statistics across the world.
Started by the International Agency for Research on Cancer in 2012, the GLOBOCAN project data revealed that there were approximately 1.8 million new lung cancer diagnoses worldwide. This accounted for nearly 13% of the global cancer burden. In this study, lung cancer remained the most common cancer diagnosis in men, with approximately 1.2 million cases in 2012. While the incidences of lung cancer vary, the highest incidence rates occur in Central and Eastern Europe and Eastern Asia with about 50/100,000, and the lowest rates in Central and Western Africa with about 2.0/100,000. The highest incidence rates in women were observed in North America with about 34/100,000 and Northern Europe with about 24/100,000, while the lowest rates are found in Western and Central Africa with about 1/100,000.[3] In the U.S., the chances that a man will develop lung cancer in his lifetime are about one in 15, and for a woman, it is about one in 17. In addition, black men are about 20% more likely to develop lung cancer than white men, but the rate is about 10% lower in black women than in white women.[4] This large variability between regions is predicted to be from the different risk factors; the people of that area are exposed to. In more developed societies, it is also much more common for people to have access to the most notable carcinogens that lead to the development of lung cancer.
With the high rate of incidence, it is a priority to find all the risk factors for lung cancer and limit exposure to them. While much research has gone in to confirm the most common causes including age, smoking tobacco, secondhand smoke, and history of chronic obstructive pulmonary disease, there are also many other causes that go largely overlooked. These other causes consist of radon exposure, occupational exposure to asbestos, arsenic, diesel exhaust, air pollution, and chromium.[5] There is also conflicting evidence about exogenous hormones among women, diet, and body mass index that may play a role in the development and progression of some types of lung cancer.[6-12] More research needs to be done to elucidate the mechanisms about how these factors play a role in oncogenic progression. This may help prevent the development of the disease or allow us to screen patients who are most at risk for early diagnosis.
Early-stage lung cancer does not typically present with symptoms that would prompt a doctor’s visit, which is why many times when a patient is diagnosed with lung cancer, it is in Stage III or IV and has metastasized to cause more systemic issues. However, the most common symptom includes a cough that does not go away and shortness of breath. In addition, some patients may experience chest pain, blood in sputum, weight loss, and more. Other times, there are no symptoms at all.[13] In late-stage patients, fewer than 10% of those diagnosed survive for more than 5 years. This may partly be due to longer time between the onset of symptoms and the patient coming to the hospital for diagnosis. This leads to more late-stage diagnoses and therefore less eligibility for potentially curative treatments dues to resistance.[13] With the current treatments for lung cancer, many types of pathways are implicated in the resistance mechanisms. These specific genes/proteins include aberrant heat shock proteins (HSP) expression and Multidrug resistance protein 1 (MRP1), Metallothionein 1G (MT1G),RAS association domain family protein 1a (RASSF1a),Kirsten rat sarcoma virus (KRAS), or G protein-coupled receptor 56 (GPR56) mutations. In this article, we discuss the current standards in the diagnosis and treatment of lung cancer as well as potential targeted therapies in these chemotherapy resistance pathways.
DIAGNOSIS
For both types of lung cancer, SCLC and NSCLC, the international staging system is used. The stages range from occult or hidden stage to Stage IV, and each increasing stage means an increase in cancer size, the number and location of metastases, and a correlated worse prognosis. The diagnostic workup for lung cancer must include confirmation of the diagnosis on examination of histology, evaluation the stage or how far the cancer has spread, and an analysis of the patient’s functional status.[14] Before performing any invasive procedures, imaging is always performed first. Common imaging studies include a magnetic resonance imaging or computed tomography scan. The findings of these scans of the chest and clinical presentation usually allow for a tentative differentiation between SCLC and NSCLC. In patients suspected of having NSCLC, the method of diagnosis is usually governed by the expected stage of the disease. Sample biopsies can be recovered by fine-needle aspiration, endoscopic ultrasound, bronchoscopy, thoracoscopy, mediastinoscopy, or a lymph node biopsy. Patients with suspected lung cancer who present with a pleural effusion can also undergo thoracentesis. The reason for this is to differentiate between a malignant effusion and a paramalignant effusion which alters the staging and potential therapies for that patient.[15] Histological confirmation is very effective in directing the most appropriate treatment, so it is carried out in all possible cases. However, in situations in which it is impossible to obtain a biopsy, an unambiguous cytology result is considered an adequate secondary option.[14] Bronchoscopy allows confirmation of the primary tumor with a sensitivity of 0.88 for central tumors and 0.78 for peripheral tumors. It also provides information for T and N-staging.[15] Based on these figures, current standard of practice is a histological confirmation from a lung specimen. This is preferred if it can be done without running excessive risks. The initial evaluation and workup must always be governed by the risk involved in the planned workup in addition to the quality of life that treatment would achieve. Cardiovascular limitations, as well as liver and renal function, are also evaluated. Specifically, in NSCLC, the most important aspects to deciding the correct type of therapy depend on staging and accurate evaluation of the patient’s functional status and whether adequate lung function would be expected to remain after any planned curative treatment.[14] Then, the current treatments are looked through to show which drugs show the most efficacy for the specific tumor type, while considering the proteins that it expresses. Further details on prognosis are summarized in [Table 1].
Stage | Percentage of cases | 5 year survival rate without treatment (%) | Median survival without treatment (months) | 5 year survival rate with treatment (%) | Median survival with treatment (months) |
---|---|---|---|---|---|
I | 10–15 | 45 | 48 | 65 | 100 |
II | 10–15 | 25 | 28 | 40 | 43 |
III | 25–35 | 15 | 12 | 22 | 20 |
IV | 40–50 | 2 | 6 | 20 | 18 |
CURRENT TREATMENT
Despite there being great improvements in patient survival over the last few decades in cancer treatment, there have been little improvements in lung cancer survival. The lack of improvement in lung cancer survival is largely attributed to by the time; a diagnosis is made. As previously mentioned, lung cancer is often in its advanced stage upon diagnosis so treatment options are limited.[3] However, when different patients are diagnosed, the stage at which their cancer is in determines the type of treatment that may have the most efficacy. As shown in [Table 1], Stages I and II make up 25–30% of cases of NSCLC at diagnosis. In patients that have no contraindications, surgical resection is the treatment of choice. After surgery, the difference between Stage I and Stage II are that Stage II patients also receive platinum-based adjuvant chemotherapy. However, In patients with Stage I or II tumors who cannot undergo surgery, radiotherapy with curative intent is the most common therapy.[14]
Stage III treatment is broken down into Stage IIIA and Stage IIIB. Stage IIIA makes up about 15–20% of all NSCLC at diagnosis. These tumors are often treated like Stage I and II non-small cell lung cancer, except in the presence of certain contraindications on a case-by-case basis. After removal of the tumor, it is followed by adjuvant chemotherapy. In some scenarios where this cancer is found in N2 lymph nodes, surgery and post-operative adjuvant platinum-based chemotherapy should be given and radiotherapy should be considered. In other situations, radio chemotherapy is recommended. However, the treatment regimen is determined on a case by case basis, taking the functional status of the patient into consideration.[14] In comparison, Stage IIIB makes up a slightly less amount of NSCLC at the time of diagnosis at about 10–15%. At this stage in the progression of NSCLC, radio chemotherapy treatment is the standard of treatment. The only variability is in reference to whether the patient is given the treatment sequentially or simultaneously. If the patient has a poor functional status, it is preferred to give them sequential therapy, but if they are in relatively good health, simultaneous treat is more advantageous.[14]
The final stage, Stage IV, consists of 40–50% of all NSCLC at diagnosis. In these patients, only palliative care is the standard treatment. The current combination of platinum with a modern combination partner, such as taxanes, gemcitabine, or vinorelbine, leads to survival times of up to 10 months. This regimen of chemotherapy has also been shown to not only lengthen lives, but also improves symptoms in most patients. With the advanced stage, there are a lot fewer effective options for treatment, but recently there has been an increasing amount of individualization in treatment regimens, summarized in [Figure 1]. One of the newest treatments is monoclonal antibodies against oncogenic-related genes, such as vascular endothelial growth factor, anaplastic lymphoma tyrosine kinase gene, epidermal growth factor receptor (EGFR), and programmed death ligand-1. All of these types of drugs have shown great efficacy and have been shown to increase overall survival in patients with Stage IIIB/IV NSCLC to over 12 months.[14] However, more research needs to be done to fully investigate when these drugs are appropriate. Over the past decade, several new agents have become available for the treatment of metastatic non-small-cell lung cancer. These drugs include the taxanes, gemcitabine, and vinorelbine.[1] The combination of one or more of these agents with a platinum compound in Phase 2 studies has resulted in high response rates and prolonged survival at 1 year.[16-19] However, since this time, there have been only a few comparisons of the newer chemotherapy regimens, which are now used frequently, with each other. In addition, due to the difference in cell origins between SCLC and NSCLC, many treatments show different efficacy when given to each. Due to this, the following findings are specific only to NSCLC.

- Treatment algorithm for non-small cell lung carcinoma. This is a step-by-step treatment protocol that is like what physicians use to guide them in choosing the most appropriate treatment. The TNM system, by the American Joint Committee on Cancer, is used for determining the stage of the tumor. When in later stages (III, IV) physicians then use gene-targeted therapy to determine aberrations in the specific cancer cell. These aberrations then help the physician predict the most efficacious drug/drugs (shown at the bottom).
One research team, The Eastern Cooperative Oncology Group, conducted a randomized and clinical trial comparing four of the platinum-based drugs. They used two-drug chemotherapy regimens in more than 1100 patients and found the median survival was 8 months, with no significant differences in overall survival among each group. This goes to show that although modest progress has been made with the use of chemotherapy in the treatment of NSCLC, additional therapeutic options are needed.[1] In advanced NSCLC, responses to these types of therapy are brief. When these drugs fail, second-line chemotherapy with docetaxel can prolong survival after platinum-based therapy. However, if this drug fails to cause a response. there is at present no defined role for third-line or fourth-line chemotherapy, as their response rates have been seen to be 2.3% and 0%, respectively.[20] Other studies show similar results that among patients that were treated with docetaxel after the failure of at least two chemotherapy regimens, survival was basically identical to that among patients treated with only supportive care.[21] This indicates that after resistance to the second-line chemotherapy, continued treatment of a third drug should be reexamined to insure that is in the best interest of the patient. There are many studies that examine many mechanisms for this resistance and one may be HSP.
HSP IN NSCLC
HSPs are a large family of chaperones that are involved in protein folding and maturation of a variety of other intracellular proteins. They aid in their folding and stability by protecting them from degradation, oxidative stress, hypoxia, and thermal stress. These proteins are also one of the most recent drug resistant mechanisms of interest in cancer cells. Interestingly, tumor cells have been found to be more HSP chaperone dependent than normal cells. This is mainly since the oncoproteins in cancer cells are often misfolded and require chaperone proteins to correct their orientation. This has led to the recent development of inhibitors of these proteins, like Hsp90. These drugs have shown promise both pre-clinically and clinically in the treatment of certain cancer cell lines, including lung cancer.[22]
POTENTIAL THERAPEUTIC GENE TARGETS
MRP1 mutations
About 25 years ago, the knowledge of the initial response rate of NSCLC tumors being much lower than SCLC to chemotherapies lead to the belief that these NSCLC displayed inherent drug resistance. The molecular basis of drug resistance in both SCLC and NSCLC remains to be poorly understood. However, the first protein examined to play a role in this mechanism was P-glycoprotein. The overexpression of this transmembrane transport protein had been detected in different multidrug-resistant tumor cell lines and in a variety of tumors with both acquired and inherent drug resistance, but despite the widespread occurrence of drug resistance in human lung tumors, overexpression of P-glycoprotein is infrequent. This leads researchers to examine other potential targets that induce alternative resistance mechanisms.[23] In a study years later, MRP1, encoded by the ABCC1 gene, was examined. It was originally discovered as a cause of multidrug resistance in tumor cells, and since then, MRP1 serves a role beyond that of only mediating the ATP-dependent efflux of compounds from cells. The other roles that this protein plays are causing efflux of both antioxidant glutathione and the pro-inflammatory cysteinyl leukotriene C4. Additional lipid mediators are also expected to be substrates of this transporter, but more research needs to be done. This multifaceted role played by MRP1 is why it likely has a role in the etiology and progression of many diseases.[24] One disease of particular interest is NSCLC.
On further examination of this protein, human MRP1 has been shown to be amplified at least 100-fold in the multidrug-resistant lung cancer cell lines. This is of particular interest because of the diversity of compounds that this protein is able to efflux.[24] Therefore, elevated MRP1 protein and messenger ribonucleic acid (RNA) levels have been considered in many tumors as predictors of poor response to chemotherapy. In another study, it was shown that the MRP1 is intrinsically expressed and functionally active in NSCLC, and this expression has an indirect correlation with the cell’s sensitivity to chemotherapeutics. In addition, almost all NSCLC cells in this study had an intrinsic MRP1 overexpression in the tumor tissue.[25] However, there is contradictory data saying that this is not always the case.[26,27]
It was proposed that the discrepancy might be explained by the detection methods used or the investigated patient groups. Whether they looked at RNA versus protein or early versus late stage of the disease may change the amount of the specific marker expressed. In this study the data showed that MRP1 expression in the malignant tissues was highest in early-stage and declined with progression into the late-stage of the cancer. This suggests that the MRP1 overexpression may be a result of cancer transformation of normal cells and then the cancer cells down regulate it as it progresses. Similarly, it was shown that MRP1 overexpression was associated with tumors that were lower grade. While the prognosis may be assumed to be better, this association was absent. The reason for this is that although these cells may be less aggressive tumors, it was found that these cancer cells are also much more resistant to chemotherapy.[25] This finding has previously been found in other studies showing that a worse prognosis of NSCLC patients was seen in patients expressing high levels of MRP1 and treated by MRP1-related exported chemotherapies.[27,28] If there is a general overexpression of this protein in early stages of the cancer transformation, this would suggest that MRP1 may play a major role in the intrinsic drug resistance of many NSCLC cell lines. It has also been hypothesized that exposure to chemotherapy may also activate the multi-drug resistance 1 (MDR1) gene and leading to an even more resistant phenotype.[25] For this reason, MRP1 is a protein that must be learned more about and drugs are being developed to target mutations associated with it.
Overview: MT1G, RASSF1a and GPR56 mutations
While a large amount of research has been done on exporters and the potential roles they play in chemotherapy resistance, another mechanism has been proposed. Changing in gene expression is not new to the development of cancer, but a new mechanism of causing the change is related to hypermethylation of genes. Many studies have shown that deoxyribonucleic acid (DNA) is much more vulnerable to methylation than alterations or mutations to the actual DNA sequence in response to external stimuli, and it is these alterations that may lead to the beginning stages of oncogenic progression. It has also been reasoned that these changes may influence the direction of the specific transformations of these cells.[29] This mechanism has already been shown in a specific adenocarcinoma of the breast, neuroblastoma cells, ovarian tumors, and colon tumors, which allows for consideration of the same mechanism occurring in the resistant progression of adenocarcinoma of the lung. In one study, a total of 3617 gene promoters were found to be differentially methylated. However, 1581 were hypermethylated and 2036 were hypomethylated in A549/DDP cells compared with A549 cells. Of these, three potential hypermethylated gene promoters were of interest. These genes were GPR56, MT1G, and RASSF1 and they were chosen because of their previously discovered role in tumorigenesis, tumor growth, or induced modulated expression to stress.
RASSF1A mutations
RASSF1 is a recently discovered RAS family-related gene that plays a role in multiple apoptotic and cell-cycle checkpoint pathways. It also has been shown to play a role in microtubule stability, most notably during sister chromatid segregation. In addition, an isoform of RASSF1, RASSF1A, is a tumor suppressor gene whose inactivation can lead to many types of cancer.[30] Its mechanisms is largely unknown, but it is believed that it may regulate the pro-apoptotic kinase MST1 which plays a major role in modulating the Hippo signaling pathway.[31] RASSF1 has also previously been examined to determine the progression and prognosis of NSCLC.[30] This is such a notable marker because loss of at least one RASSF1A allele was found in more than 90% of small cell lung cancer and in 50–80% of non-small cell lung cancer.[32] RASSF1A ability to stabilize tubulin has been compared to the taxol drug family. In a standard cell, if the microtubule spindle complex is not formed properly, the cell will undergo apoptosis. This is to prevent mutations and aneuploidy. When this does not occur, chromosomal missegregation and inheritable aneuploidy arises, leading to the chance of malignancy.[30] This process has been observed in the previous studies. In a study on mouse embryonic fibroblasts, Rassf1a/mice were bread and allowed to mature. While Rassf1a/ mice were viable, by the average age of 14 months, all of the models had an increase in tumor incidence.[33,34] With this data, it can be concluded that RASSF1A has a tumor suppressor function that needs to be investigated.
MT1G
MT1G belongs to a class of metal binding proteins, and its roles are related to its metal binding property. These including the detoxification of heavy metals and inhibiting their accumulation, donation of zinc and copper to enzymes and transcription factors, like nuclear factor kappa B (NF-κB), and protecting the cell against oxidative stress. Like RASSF1, MT1G has also previously been examined to determine the progression and prognosis of NSCLC.[30] While the current incidence of mutated MT1G is not known in cancer cells, the previous studies showed that MT1G expression was repressed by methylation of the promoter region in several human cancers, including hepatocellular cancer, colorectal cancer, prostate cancer, and thyroid cancer.[35-38] In addition, restoration of the down regulated MT1G expression in some cancer cells inhibited cell growth both in vitro and in vivo. This suggests that this protein may play a role as a tumor suppressor gene, but its mechanism is unknown.[39] However, based on one study, ectopic expression of MT1G in certain cancer cells dramatically inhibited cell growth and invasiveness. It did so by inducing cell cycle arrest and apoptosis. By modulating the activity of phosphatidylinositol-3-kinase (PI3K)/Akt pathway, these cells showed decreased expression of Mdm2 allowing p53 to carry out its tumor suppressing function.[40] However, other research has shown contradicting information.[41] Hence, more research needs to be done to elucidate the role of MT1G in oncogenic progression and chemotherapy resistance.
GPR56
GPR56 is a G protein-coupled receptor that has been shown to be implicated in brain development and plays a role in cancer progression by inhibiting tumor growth and metastasis. While this has been seen in human melanoma cell line xenograft models, its role in MDR of lung cancer is still unknown. In the study on human melanoma cells, expression levels of this protein were also found to be inversely correlated with metastases. It was also shown that its re-expression led to suppressed melanoma growth and metastasis in a xenograft cancer model.[42] However, in other studies, the expression levels of GPR56 were reportedly increased in cancerous lesions compared with those in adjacent normal tissues in several cancer types.[43-45] This led to the idea that GPR56 might play an inducing role at the early stages of cancer development, but decrease in expression as the tumor cells progress to a more undifferentiated state.[42] Because the mechanism of GPR56 is unknown in the regulation of cancer, multiple lines of evidence suggests that GPR56 functions as an adhesion receptor. It is also believed to interact with the extracellular matrix (ECM) directly. The reason that this is indicated is because it has been shown to bind to TG2, a major cross-linking enzyme of the ECM.[46,47] While this study found no major structural differences between normal models and GPR56−/−models, the mechanism of GPR56 in cancer may lie more with its association to TG2. TG2, affects fibronectin-mediated cell adhesion through integrins in an enzyme-independent manner and with a loss of adhesion metastases may be more common, but more research needs to be done on this mechanism and how this protein may cause chemotherapy resistance.[42]
KRAS
KRAS is a small membrane bound GTPase that has been found to be in 20–30% of lung adenocarcinomas in western countries. With a gain of function mutation, this protein has been shown to induce tumorigenesis and rapid cellular growth. In the proteins normal structure, it is switched from the active and inactive form by guanine nucleotide exchange factors and GTPase-activating proteins. In the presence of GTPase-activating proteins, the hydrolytic activity of KRAS is increased significantly. GEFs cause the release of GDP. This release allows free cellular GTP to bind to RAS, reactivating it. The mutations that are of significance to tumorigenesis are ones that impair the ability of KRAS to hydrolyze GTP. With this loss of function, KRAS is locked in a constitutively active state. The downstream signaling cascades remain on leading to uncontrolled cellular proliferation and survival. Another interesting aspect of KRAS mutations is that specific mutations have been shown to have different biological behaviors and subsequent different clinical outcomes. This is likely due to the protein’s change in affinity for the cofactors and the overall activation of the downstream cascade. This mechanism is understood to be the way these tumor cells develop resistance to regular chemotherapy. One key component of KRAS signaling is activation of mammalian target of rapamycin (mTOR). This protein plays a direct role in bypassing the regulatory mechanisms of the cell that many chemotherapies drugs target. With the continuous activation of the cascade, mTOR accumulates and causes normal chemotherapy agents to be much less efficacious.
HSP90
HSPs are highly expressed proteins that consist of >2% of all cellular proteins and play a role in stabilizing molecules of the cell in response to stress. It is also known for helping orient proteins into their proper tertiary structure. This function plays an important role in numerous biological functions including cell survival, proliferation, cancer progression, and metastasis.[48] Originally, HSP was thought to be only found inside of cells, but these proteins now have been discovered to have cytokine like properties in the extracellular space. Several types of HSP, including Hsp60, Hsp72, and Hsp90, have been found high concentrations outside of cells after cellular damage and necrotic release or secreted in response to certain stressful stimuli.[49] These extracellular HSP is thought to play an important role in modulating the immune system, specifically in response to an infection or a disease.[49] The modulating role is in response to HSP ability to binding to specific peptides. These peptides act as the fingerprint of the cause of the cell death and its origin. These markers are then recognized by the immune system.[50] The immune recognition of these proteins relies on antigen presenting cells (APCs). The chaperoned peptides are transferred to APC to induce CD8+ T lymphocytes specific for that peptide. This antigen-specific immunity is like other immunogenic mechanism seen through the body. The other role HSP play in modulating the immune system is their ability to induce stimulation of pro-inflammatory cytokine[51] and chemokine production.[52] These cytokines are non-specific in regulating immune responses, so extracellular HSP plays a critical role in activation of both the innate and the adaptive immune systems. This dual role of HSP as chaperones and cytokines is now referred to as chaperone activity.[53,54]
What is unique about HSP and cancer is that the normal level of expression changes when a cell undergoes transformation into a tumor cell. It has been shown that these cells will increase their expression anywhere from two to tenfold.[55,56] This higher expression has also been linked to a higher risk of recurrence, distant metastasis, and a poorer prognosis.[57] It does so because of its close ties to the Janus kinase/Signal transducer and activator of transcription (JAK/STAT) pathway as well as many other tumor transforming proteins. Hsp90 is essential for the function of STAT3 [Figure 2] and this pathway is what controls the tumor microenvironment and cancer progression.[48,55,58] Hsp90 has also been shown to stabilize PI3K and AKT proteins. It may also be through this mechanism in which Hsp90 overexpressing cells may be inhibiting cellular apoptosis in tumors.[48] Overall, there has been a large correlation with HSP, but more research needs to be done to explain the exact role in which this protein plays in tumor development and chemotherapy resistance.

- Heat shock proteins 90 (HSP90) pathway and HSP90 inhibitor’s role in cancer progression. This is a general overview of the different pathways that Hsp90 plays a role in. Hsp90 (dark green) binds to multiple different proteins (labeled stabilized signaling protein) that go on to act as receptors, signaling molecules and more. When bound together, Hsp90 stabilizes the signaling protein allowing it to properly orient itself in an dependent manner. If this process runs unchecked, it can cause aberrant gene expression through multiple pathways and sequential tumorigenesis. However, if exposed to an Hsp90 inhibitor these proteins will no longer orient properly and deplete some of the cell’s ATP. When incorrectly folded or unbound to Hsp90, Ubiquitin E3 (Red) binds to the signaling protein and adds ubiquitin molecules (Ub) to it. This causes programmed protein degradation and downregulates the cancer/resistance causing pathways.
DRUGS OF INTEREST
Aminoxyrone
As previously mentioned, Hsp90 plays a major role in the stability of proteins that can have oncogenic effects. One is the protein STAT3, and in the previous studies, the constitutive activation of STAT3 in breast cancer was associated with drug resistance to chemotherapy and a worse prognosis. However, blockage of the JAK/STAT axis elicits antiangiogenic and antimetastatic effects through suppression of the downstream signaling pathways. Studies show that a direct interaction of STAT3 and Hsp90 occurs before STAT3’s interaction with JAK. Therefore, an Hsp90 inhibitor may provide a means of treatment for drug resistant tumors with overactivation of the JAK/STAT pathway or abnormalities from an overexpression of Hsp90.[55,58] It may also play a role in down regulating other molecular targets that help tumor progression and proliferation outside of the JAK/STAT axis [Figure 2]. While many trials have been performed, all HSP inhibitors have shown high levels of toxicity. The reason for this is because they induce a heat shock response. While many treatments have been developed and put through clinical trials, all have been stopped and there are no Food and Drug Administration (FDA) approved HSP inhibitors used in the treatment of NSCLC. In addition, a cell also has multiple redundancies to HSP, and when these older generation HSP inhibitors inactivated Hsp90, the cell would retain its resistance.[55] However, with the drug Aminoxyrone, this response no longer takes place. As of now, all older generation Hsp90 inhibitors bind to the ATP binding site in the N-terminal domain of the Hsp90 protein. While this has been shown to inhibit Hsp90, this also caused the heat shock response, activating Hsp70, Hsp40, or Hsp27. These proteins with their redundant or compensatory antiapoptotic function maintained the stability of the mutated proteins and preventing cell death. Aminoxyrone however utilizes a different mechanism. It prevents dimerization of Hsp90 protein in the C-terminal region of the protein. This inhibited Hsp90’s action and did not result in the heat shock response.[59] However, no clinical trials have been done to determine the efficacy and safety of this drug in patients, so more research is needed.
Pan-class I PI3K inhibitor: Copanlisib
The PI3K/AKT/mTOR signaling pathway is one of the most important and well-known regulators of cell growth, motility, survival, metabolism, and angiogenesis.[60,61] In addition, activation of this pathway contributes to the development of tumors and resistance to chemotherapies,[62] but inhibition of PI3K can decrease cellular proliferation and increased cellular death.[63] The inhibition of PI3K can be done by multiple small molecule inhibitors, but one of particular interest is the pan-PI3K inhibitors Copanlisib. In normal physiologic conditions, PI3K is activated by extracellular stimuli, such as growth factors, cytokines, and hormones. Once it is activated, PI3K can catalyze the phosphorylation and produce phosphatidylinositol molecules which binds and recruits a number of other intracellular signaling molecules, one of which is AKT which activates cell growth and cell survival pathways.[64] In the previous studies, mutations of these kinases or decreased expression of their regulatory pathways have led to oncogenic transformation. This showed its central role in increasing tumor vasculature and invasive potential of cancer cells. Deregulation of this pathway can come from many mechanisms because of the diverse number of molecules involved, but the most well-known include the loss or inactivation of the tumor suppressor PTEN, mutation, or amplification of PI3K, or activation of oncogenes upstream of PI3K. Copanlisib (BAY 80-6946) is a highly selective and reversible pan-class I PI3K inhibitor. Its main activity is against the signaling molecule isoforms p110α and p110δ, and it is currently in clinical development.[65] At present, multiple Phase II trials are underway, and one trial with patients suffering from relapsed or refractory indolent B-cell lymphoma had such promising results (overall response rates of 59% and complete response rates of 12%) that it leads to the accelerated approval of Copanlisib for relapsed follicular lymphoma.[66] Clinical trials of Copanlisib are also in patients with non-Hodgkin lymphoma, but no treatment outcomes have been done on any cell lines of patients with NSCLC. However, with these promising results, it is worth examining how this drug may play a role in inhibiting the aberrant expression of the PI3K/ Akt pathway in down regulated MT1G cancers cells.
Demethylation drugs: Norcantharidin (NCTD) and 5-Aza-CdR
Gene expression is controlled in multiple ways in eukaryotes, but methylation of cytosine bases in DNA is a common epigenetic manipulation that cells use to turn genes off. This epigenetic change occurs at CpG islands in the promoter regions, and the enzyme responsible for this is DNA methyltransferase. Studies have shown that this mechanism is important in embryonic development, genomic imprinting, X-chromosome inactivation, and chromosome stability and because this mechanism is of high importance, it is no surprise that the overall methylation of promoters is similar in the same tissue from person to person. However, there have been significant differences found between normal and cancer tissues from the same location. While tumor suppressor genes are often silenced in cancer cells due to hypermethylation, the genomes of cancer cells have also been shown to be hypomethylated overall when compared to normal cells.[67] This is expected in cancer cells because low methylation of DNA can cause chromosomal instability, which may result in chromosome breakage, translocations, and oncogene activation.[32] How the cell determines what genes are to be methylated is unknown, but present research is being done to elucidate these mechanisms. In the past, methylation was observed using immunofluoroscopy against 5-methylcytosine, but as of recent, processes such as matrix-assisted laser desorption/ionization time-of-flight mass spectrometry (MALDI-TOF-MS) have become more common.[67]
NCTD has been shown to play a role in inhibiting many types of cancer, but its role in mechanism is not completely understood. In one study, it was shown that NCTD had the ability to decrease cyclin D1. It was proposed that it did so by targeting the PI3K/Akt/NF-κ signaling pathway. Results of this study also showed that NCTD downregulated the expression of anti-apoptosis proteins, such as survivin, XIAP, cIAP1, and B-cell lymphoma-2, and upregulated the apoptotic protein BAX.[68] In another study, NCTD was able to reverse the methylation state of RASSF1A gene and induce its re-expression causing arrest at G2/M phase and apoptosis in hepatocellular carcinoma cells. RASSF1A has also been implicated in cisplatin resistant adenocarcinomas which makes it a specific gene of interest.[69] This finding provides a reason to evaluate what other genes this drug may also demethylate. Similarly, in one last study, NCTD had also shown the ability to reverse MDR human breast cancer cells by regulating the sonic hedgehog signaling.[70] At present, this drug has no indications or clinical outcomes for any types of cancers because it is not FDA approved in the United States, but because of its promising results in multiple cell lines, it may be a promising therapeutic in cancer cells suppressing gene expression through hypermethylation.
5-Aza-CdR acts as DNA methyltransferase inhibitor. In cells with hypermethylation, this drug has been shown to modify suppression of genes important for differentiation and proliferation. What is unique about this drug is that it is insensitive to non-proliferative cells. At present, the Federal Drug Administration has approved DNA methyltransferase inhibitors for the treatment of myelodysplastic syndrome, hematological malignancies, and to reduce the malignant potential of solid tumors. This drug has been shown to cause cell apoptosis and alter cell growth. However, 5-Aza-CdR is not very stable and has shown some toxicity in aqueous solution.[32] There also are no current indications for NSCLC, but it does show promise in cancer cells suppressing gene expression through hypermethylation.
MRP1 inhibitor: Reversan
Reversan has been identified as an MRP1 inhibitor that is safe and does not affect the pharmacokinetic properties of other therapeutic agents. The reason that this is notable is because other drugs targeting similar proteins bound the exporter and competed with other therapeutic agents for transportation in addition to CYP3A4. This is important because it was by this mechanism that this drug caused high toxicity. Studies have shown that Reversan is not a substrate for MRP1 by demonstrating that there are similar accumulations of the drug intracellularly in different cells, despite the increased level of MRP1 in the different cells due to gene amplification. At present, the exact mechanism of action of Reversan is unknown, but since the original exporter modulators were not ATPase inhibitors, it is likely that this drug has a similar mechanism. However, this remains to be tested. It has also been theorized that Reversan does not directly interact with MRP1, but it rather alters the properties of the membrane around the transporter. It is believed that this type of mechanism would then alter the structure of the protein and change its ability to export drugs.[71,72] Even though Reversan inhibits the function of multiple drug exporters, it does not significantly alter the toxicity profile of conventional chemotherapeutic relative to other exporter inhibitors. This may be explained by the fact that Reversan does not interact with CYP3A4 like other inhibitors that have been shown to do. Because of this effectiveness in the absence of increased toxicity of other drugs, Reversan has potential for an excellent therapeutic index compared to other similar drugs in the same family when targeting cancers associated with MRP1 or other multidrug transport overexpression.[71,72] This drug is in the testing phase of multiple cancers and has no indications for NSCLC yet. Promising results were shown in other types of cancers in the Phase I clinical trial performed by the Roswell Park Cancer Institute Corp.
KRAS inhibitor: AMG 510/Sortorasib
In the past, KRAS-mutant lung cancer, the treatment options were still limited, and chemotherapies remain the first-line recommendation. However, recent developments show promise in therapy with much better efficacy. In the previous studies, the presence of KRAS mutations has shown mixed findings on prognostic and response to chemo and targeted therapy which made choosing first line treatment difficult. Since that time, AMG510 has since been developed and has shown significant improvement in reducing tumor size and decreased disease progression, especially when combined with other chemo and targeted therapies. This drug works by binding to the P2 pocket of KRAS, inhibiting its continuous activation and downstream effects. It has also been shown that this drug is more than 20 times more potent than any other drug in its class. This drug is currently in clinical trials (NCT03600883) and showing promising results. In two patients in the trial, tumors have had a decrease in 34% and 67% with a median progression free survival of 6.9 months for all the patients. In addition, the side effect profile was shown to be limited to gastrointestinal upset and mild liver enzyme elevation or damage with only 2% of patients in the trial having serious side effects having to stop treatment. While this drug is not currently FDA approved for NSCLC treatment, this drug has shown its potential in being a great adjunct therapy for resistant NSCLC, but further testing needs done to determine in which patients and diseases the treatment will be most efficacious and safe.
MALDI-TOF technology
MALDI-TOF-MS is a new device for proteomic analysis. It is state of the art and is chosen of other protein analytics devices due to its sensitivity, fast turnover, low cost, and ease of use. It is also able to run multiple different tissue samples, making it one of the most diverse analytic devices used today. On running a sample, peaks correspond to ions formed from relatively abundant compounds in the sample. In a majority of samples, this consists mostly of peptides or proteins, but samples can also be run to examine them for something as diverse as the presence of microbes or the presence of methylated genes.[73] One of the most notable uses was that of peptide mass fingerprinting. This is important because this information can be used to detect diagnostic, prognostic, and predictive proteomic biomarkers. In addition, MALDITOF MS has also been using this fingerprinting to guide the development of algorithms to decide the efficacy of certain treatments. This is done by comparing patients with similar diseases and treatment and looking at the protein markers that make up the cell. One example of this can be seen in the development of the algorithm looking into the efficacy of EGFR-tyrosine kinase inhibitor (TKI). Based on the associated mutations of certain cells, this program has been able to help guide treatment of patients that may benefit from the EGFR-TKIs.[74,75] In this study, we aimed to detect serum proteins that differentiate the A549 and A549/ DDP cell lines and may be causing cisplatin resistance. Once we had determined the genes of interest, we targeted those genes either directly or through associated pathways. We then compared how each cell responded to the therapy by re-examining the target gene expressions. Throughout this project, we were looking to see if targeting these genes were able to sensitize the resistant cells to chemotherapy to guide future treatment.
FUTURE TREATMENT
Over the past few decades, targeted therapy and immunotherapy have made great contributions to the treatment of lung cancer. Targeted therapy has been used to use small molecular inhibitors to block the function of mutated proteins of the tumor while immunotherapy uses the idea of boosting the body’s own immune system to destroy the cancer cells specifically. While each of these treatments has both advantages and limitations, the overarching problem with all cancers is resistance. With the new use of MALDI-TOF-MS, therapies are now able to be prescribed only if they show efficacy. In addition, with this device, we are also developing drugs that can halt the cell’s ability to resist treatments and make current treatments more efficacious. All of this research is extremely important because lung cancer remains to be the greatest cause of death from cancer in the United States.[2]
DISCUSSION
NSCLC currently is the leading cause of death among all cancer types with < 10% of patients have a 5-year survival rate.[1,13] With 1.8 million new lung cancer diagnosis worldwide, this accounted for nearly 13% of the global cancer burden in 2012 and these numbers have only increased since making lung cancer the most common cancer worldwide.[3] Depending on staging, surgery, chemotherapy, and radiotherapy are current standards of treatment with more research going into small molecule inhibitors and immunotherapies.[14] While it has been shown that these combinations have had positive responses, some tumors have developed resistance to general chemotherapies such as cisplatin, which shows the importance of understanding the mechanisms behind this acquired resistance.
Aminoxyrone is an Hsp90 inhibitor, and unlike other HSP inhibitors, this drug shows significantly less toxicity levels while showing equivalent inhibitory responses. The reason for this is because Aminoxyrone does not cause a heat shock response.[55,59] In addition, NSCLC has been shown to have overexpression of Hsp90 and HSP are largely correlated with developing resistance to cell death and resistance to chemotherapies. Hsp90 specifically plays a role in the JAK/ STAT pathway and aberrant expression has been shown to cause cell growth and survival.[55,58] Although not currently FDA approved for adjuvant NSCLC therapy, with the high expression of Hsp90 found in NSCLC, this is a drug of interest in reversing the resistance of tumor cells.
Copanlisib is a reversible pan-class I PI3K inhibitor selective for p110α and p110δ. MT1G has been shown to be silenced in cancer cells due to hypermethylation. With this gene silenced, other pathways have been shown to be overactive and unregulated such as the PI3K/AKT/mTOR signaling pathway. This pathway is one of the most important regulators of many cell proliferative processes; therefore, tumor progression as well as chemotherapy resistance.[60,61] Although this drug is not currently FDA approved for NSCLC, because it can inhibit this aberrant pathway, further research needs to be done to see the synergistic effects, it may have when paired with other chemotherapy sensitizing therapies.
NCTD has been shown to have a diverse array of possible antitumor mechanisms, but its exact reach is not known. The most current studies have shown that this drug plays a role in both inactivating the PI3K/Akt/NF-κ signaling pathway as well as expressing previously silenced genes by decreasing their promoter methylation. The most interesting re-expressed protein is RASSF1A as it has been shown to be downregulated in cells showing resistance to chemotherapy. Moreover, while this drug is not FDA approved in the U.S., it has been shown to have promising results in other countries. 5-Aza-CdR has a similar role to NCTD in inhibiting DNA methylation. This drug is unique because it is insensitive to non-proliferative cells.[32] At present, the FDA has approved DNA methyltransferase inhibitors, but there also are no current indications for NSCLC although it may have a therapeutic effect. Because of this, NCTD and 5-Azr-CdR are unique and exciting new drugs that may have synergistic effects with other drugs to resensitize cancer cells, particularly ones suppressing expression of regulatory genes through hypermethylation.
Reversan is a known inhibitor of MRP1 protein which has been shown to play a major role in chemotherapy resistance. This protein’s role in resistance is that it could export drugs from target cells and allow them to decrease their toxicity. While there have been many drugs developed to target this mechanism, Reversan is of unique interest because it has decreased toxicity compared to other drugs in its class because it does not use CYP3A4. While the exact mechanism is unknown or approved in the United States for NSCLC, this drug has potential for a high therapeutic index relative to the other drugs in its class. This is why it is currently in the testing phase to examine the synergistic effect; it has with other cancers in addition to determining its ability to reverse the acquired resistance seen in many cancer types.[71,72]
Sortorasib is a newly developed KRAS inhibitor that has been shown to overcome the mechanism of resistance that many cancers have acquired to common chemotherapy agents. Through the downstream cascade of mTOR, this drug has been able to reverse the replicative properties of the tumor cells and allow the organism’s cell checkpoints to properly regulate cell growth while providing the body’s immune system enough time to appropriately handle the aberrant body of cells. While there have been multiple drugs developed to target this mechanism, Sortorasib has shown much greater efficacy and a significantly smaller side effect profile. This drug shows great promise in the future of oncological treatment, but further studies need to be performed in Stages 2 and 3 clinical trials for a broader view of the effects of this therapy.
CONCLUSION
This article has discussed the current clinical standards of treating NSCLC, signal pathways of how these tumors acquire resistance, potential drug targets for these pathways, and the role HSP plays in NSCLC. With this information, the future studies could be designed to target HSP, PI3K aberrant expression, hypermethylation with gene silencing, MRP1 overexpression, and KRAS mutations utilizing combination therapies to examine the potential synergist properties to re-sensitize tumor cells. In addition, these combination therapies should also be investigated to see if they have their own anti-tumor effects blocking proliferation and survival as some of them are not currently used for cancer treatment. This would allow for repurposing of current drugs on the market or bringing to light novel therapies with more efficacy.
Acknowledgments
This work was supported in part by the Medical Summer Research Program (MSRP) (to M.A.M., N.T.N., S.M.M., S.T.M), a grant from the Mitchell Family (to A.C.M.) and institutional support from the University of Toledo College of Medicine and Life Sciences (to P.K. and A.A.).
Ethical approval for studies involving humans
This article does not contain any studies with human participants performed by any of the authors.
Ethical approval for studies involving animals
This article does not contain any studies with animals performed by any of the authors.
Declaration of patient consent
Patient’s consent not required as there are no patients in this study.
Conflicts of interest
There are no conflicts of interest.
Financial support and sponsorship
Nil.
References
- Comparison of four chemotherapy regimens for advanced non-small-cell lung cancer. N Engl J Med. 2002;346:92-8.
- [CrossRef] [PubMed] [Google Scholar]
- Non-small cell lung cancer: Current treatment and future advances. Transl Lung Cancer Res. 2016;5:288-300.
- [CrossRef] [PubMed] [Google Scholar]
- Racial and ethnic differences in the epidemiology and genomics of lung cancer. Cancer Control. 2016;23:338-46.
- [CrossRef] [PubMed] [Google Scholar]
- GLOBOCAN 2012: Estimated Cancer Incidence, Morality, and Prevalence Worldwide in 2012. Lyon, France: International Agency of Research on Cancer; 2019.
- [Google Scholar]
- Lung cancer: Epidemiology, etiology, and prevention. Clin Chest Med. 2011;32:605-44.
- [CrossRef] [PubMed] [Google Scholar]
- Reproductive and hormonal factors and lung cancer risk in the NIH-AARP Diet and Health Study cohort. Cancer Epidemiol Biomarkers Prev. 2011;20:900-11.
- [CrossRef] [PubMed] [Google Scholar]
- Body mass index and risk of lung cancer among never, former, and current smokers. J Natl Cancer Inst. 2012;104:778-89.
- [CrossRef] [PubMed] [Google Scholar]
- Fish consumption and lung cancer risk: Systematic review and meta-analysis. Nutr Cancer. 2014;66:539-49.
- [CrossRef] [PubMed] [Google Scholar]
- Cruciferous vegetables consumption and the risk of female lung cancer: A prospective study and a meta-analysis. Ann Oncol. 2013;24:1918-24.
- [CrossRef] [PubMed] [Google Scholar]
- Soy food consumption and lung cancer risk: A meta-analysis using a common measure across studies. Nutr Cancer. 2013;65:625-32.
- [CrossRef] [PubMed] [Google Scholar]
- Obesity and incidence of lung cancer: A meta-analysis. Int J Cancer. 2013;132:1162-9.
- [CrossRef] [Google Scholar]
- Hormone replacement therapy in females can decrease the risk of lung cancer: A meta-analysis. PLoS One. 2013;8:e71236.
- [CrossRef] [PubMed] [Google Scholar]
- Symptoms and other factors associated with time to diagnosis and stage of lung cancer: A prospective cohort study. Br J Cancer. 2015;112(Suppl 1):S6-13.
- [CrossRef] [PubMed] [Google Scholar]
- Lung cancer: Current diagnosis and treatment. Dtsch Arztebl Int. 2009;106:809-20.
- [CrossRef] [PubMed] [Google Scholar]
- Initial diagnosis of lung cancer: ACCP evidence-based clinical practice guidelines. Chest. 2007;132(3 Supplement):131S-48.
- [CrossRef] [PubMed] [Google Scholar]
- 1086 A Phase II study of gemcitabine with cisplatin in patients with non-small cell lung cancer. Eur J Cancer. 1995;31:S226.
- [CrossRef] [Google Scholar]
- Cisplatin-gemcitabine combination in advanced non-small-cell lung cancer: A Phase II study. J Clin Oncol. 1997;15:297-303.
- [CrossRef] [PubMed] [Google Scholar]
- Paclitaxel and carboplatin in combination in the treatment of advanced non-small-cell lung cancer: A Phase II toxicity, response, and survival analysis. J Clin Oncol. 1995;13:1860-70.
- [CrossRef] [PubMed] [Google Scholar]
- Phase II study of docetaxel and cisplatin in advanced non-small-cell lung cancer. J Clin Oncol. 1998;16:1948-53.
- [CrossRef] [PubMed] [Google Scholar]
- A retrospective analysis of the outcome of patients who have received two prior chemotherapy regimens including platinum and docetaxel for recurrent non-small-cell lung cancer. Lung Cancer. 2003;39:55-61.
- [CrossRef] [PubMed] [Google Scholar]
- Erlotinib in previously treated non-small-cell lung cancer. N Engl J Med. 2005;353:123-32.
- [CrossRef] [PubMed] [Google Scholar]
- Targeting heat shock proteins in cancer: A promising therapeutic approach. Int J Mol Sci. 2017;18:1978.
- [CrossRef] [PubMed] [Google Scholar]
- Expression of multidrug resistance protein/ GS-X pump and γ-glutamylcysteine synthetase genes is regulated by oxidative stress. J Biol Chem. 1998;273:31075-85.
- [CrossRef] [PubMed] [Google Scholar]
- Multidrug resistance protein 1 (MRP1, ABCC1), a “multitasking” ATP-binding cassette (ABC) transporter. J Biol Chem. 2014;289:30880-8.
- [CrossRef] [PubMed] [Google Scholar]
- Multidrug resistance markers P-glycoprotein, multidrug resistance protein 1, and lung resistance protein in non-small cell lung cancer: Prognostic implications. Cancer Res Clin Oncol. 2005;131:355-63.
- [CrossRef] [PubMed] [Google Scholar]
- Expression of the human major vault protein LRP in human lung cancer samples and normal lung tissues. Ann Oncol. 1996;7:625-30.
- [CrossRef] [PubMed] [Google Scholar]
- Expression of the multidrug resistance-associated protein (MRP) gene in non-small-cell lung cancer. Br J Cancer. 1995;72:550-4.
- [CrossRef] [PubMed] [Google Scholar]
- Multidrug resistance-associated protein and mutant p53 protein expression in non-small cell lung cancer. Mod Pathol. 1998;11:1059-63.
- [Google Scholar]
- Epigenetic gene silencing in cancer-a mechanism for early oncogenic pathway addiction? Nat Rev Cancer. 2006;6:107-16.
- [CrossRef] [PubMed] [Google Scholar]
- RASSF1 polymorphisms in cancer. Mol Biol Int. 2012;2012:365213.
- [CrossRef] [PubMed] [Google Scholar]
- Regulation of the MST1 kinase by autophosphorylation, by the growth inhibitory proteins, RASSF1 and NORE1, and by Ras. Biochem J. 2004;381(Pt 2):453-62.
- [CrossRef] [PubMed] [Google Scholar]
- Effect of DNA methylation inhibitor on RASSF1A genes expression in non-small cell lung cancer cell line A549 and A549DDP. Cancer Cell Int. 2013;13:91.
- [CrossRef] [PubMed] [Google Scholar]
- Tumor susceptibility of Rassf1a knockout mice. Cancer Res. 2005;65:92.
- [CrossRef] [PubMed] [Google Scholar]
- The RASSF1A isoform of RASSF1 promotes microtubule stability and suppresses tumorigenesis. Mol Cell Biol. 2005;25:8356-67.
- [CrossRef] [PubMed] [Google Scholar]
- Metallothionein expression in colorectal cancer: Relevance of different isoforms for tumor progression and patient survival. Hum Pathol. 2012;43:197-208.
- [CrossRef] [PubMed] [Google Scholar]
- MT1G hypermethylation is associated with higher tumor stage in prostate cancer. Cancer Epidemiol Biomarkers Prev. 2005;14:1274-8.
- [CrossRef] [PubMed] [Google Scholar]
- Hypermethylation, but not LOH, is associated with the low expression of MT1G and CRABP1 in papillary thyroid carcinoma. Int J Cancer. 2003;104:735-44.
- [CrossRef] [PubMed] [Google Scholar]
- Detection of metallothionein 1G as a methylated tumor suppressor gene in human hepatocellular carcinoma using a novel method of double combination array analysis. Int J Oncol. 2009;53:477-83.
- [CrossRef] [PubMed] [Google Scholar]
- Metallothionein 1G acts as an oncosupressor in papillary thyroid carcinoma. Lab Invest. 2008;88:474-81.
- [CrossRef] [PubMed] [Google Scholar]
- Metallothionein 1G functions as a tumor suppressor in thyroid cancer through modulating the PI3K/Akt signaling pathway. BMC Cancer. 2013;13:462.
- [CrossRef] [PubMed] [Google Scholar]
- The roles of metallothioneins in carcinogenesis. J Hematol Oncol. 2018;11:107.
- [CrossRef] [PubMed] [Google Scholar]
- GPR56 plays varying roles in endogenous cancer progression. Clin Exp Metastasis. 2010;27:241-9.
- [CrossRef] [PubMed] [Google Scholar]
- GPR56 promotes proliferation of colorectal cancer cells and enhances metastasis via epithelialmesenchymal transition through PI3K/ AKT signaling activation. Oncol Rep. 2018;40:1885-96.
- [CrossRef] [Google Scholar]
- GPR56 is a GPCR that is overexpressed in gliomas and functions in tumor cell adhesion. Oncogene. 2005;24:1673-82.
- [CrossRef] [PubMed] [Google Scholar]
- Differential expression of G-protein coupled receptor 56 in human esophageal squamous cell carcinoma. Cancer Lett. 2006;233:265-70.
- [CrossRef] [PubMed] [Google Scholar]
- GPR56 and TG2: Possible roles in suppression of tumor growth by the microenvironment. Cell Cycle. 2007;6:160-5.
- [CrossRef] [PubMed] [Google Scholar]
- GPR56, an atypical G protein-coupled receptor, binds tissue transglutaminase, TG2, and inhibits melanoma tumor growth and metastasis. Proc Natl Acad Sci U S A. 2006;103:9023-8.
- [CrossRef] [PubMed] [Google Scholar]
- HSP90 as a novel molecular target in non-small-cell lung cancer. Lung Cancer (Auckl). 2016;7:11-7.
- [CrossRef] [PubMed] [Google Scholar]
- Role of toll-like receptors in chaperokine-induced signaling In: Asea A, Murphy JR, Rothstein AM, eds. Emerging Role of Toll-Like Receptors in Biology and Medicine. Cambridge, MA: CRC Press; 2006.
- [Google Scholar]
- Hsp-based tumor vaccines: State-of-the-art and future directions. Curr Opin Mol Ther. 2007;9:385-91.
- [Google Scholar]
- Novel signal transduction pathway utilized by extracellular HSP70: Role of toll-like receptor (TLR) 2 and TLR4. J Biol Chem. 2002;277:15028-34.
- [CrossRef] [PubMed] [Google Scholar]
- Heat shock proteins generate β-chemokines which function as innate adjuvants enhancing adaptive immunity. Eur J Immunol. 2000;30:594-603.
- [CrossRef] [PubMed] [Google Scholar]
- Cancer immunotherapy and heat-shock proteins: Promises and challenges. Exp Opin Biol Ther. 2004;4:363-73.
- [CrossRef] [PubMed] [Google Scholar]
- Purification of recombinant and endogenous HSP70s. Methods. 2004;32:7-12.
- [CrossRef] [PubMed] [Google Scholar]
- C-terminal HSP90 inhibitor L80 elicits anti-metastatic effects in triple-negative breast cancer via STAT3 inhibition. Cancer Lett. 2019;447:141-53.
- [CrossRef] [PubMed] [Google Scholar]
- Heat shock proteins and cancer. Trends Pharmacol Sci. 2017;38:226-56.
- [CrossRef] [PubMed] [Google Scholar]
- Amplification and high-level expression of heat shock protein 90 marks aggressive phenotypes of human epidermal growth factor Receptor 2 negative breast cancer. Breast Cancer Res. 2012;14:R62.
- [CrossRef] [PubMed] [Google Scholar]
- HSP90 inhibitors in lung cancer: Promise still unfulfilled. Clin Adv Hematol Oncol. 2016;14:346-56.
- [Google Scholar]
- HSP90 inhibition without heat shock response. Blood. 2018;132:241-2.
- [CrossRef] [PubMed] [Google Scholar]
- The evolution of phosphatidylinositol 3-kinases as regulators of growth and metabolism. Nat Rev Genet. 2006;7:606-19.
- [CrossRef] [PubMed] [Google Scholar]
- Cellular function of phosphoinositide 3-kinases: Implications for development, immunity, homeostasis, and cancer. Ann Rev Cell Dev Biol. 2001;17:615-75.
- [CrossRef] [PubMed] [Google Scholar]
- PI3K/AKT signaling pathway and cancer: An updated review. Ann Med. 2014;46:372-83.
- [CrossRef] [PubMed] [Google Scholar]
- Exploiting the PI3K/AKT pathway for cancer drug discovery. Nat Rev Drug Discov. 2005;4:988-1004.
- [CrossRef] [PubMed] [Google Scholar]
- AKT/PKB signaling: Navigating downstream. Cell. 2007;129:1261-74.
- [CrossRef] [PubMed] [Google Scholar]
- BAY 80-6946 is a highly selective intravenous PI3K inhibitor with potent p110α and p110δ activities in tumor cell lines and xenograft models. Mol Cancer Ther. 2013;12:2319-30.
- [CrossRef] [PubMed] [Google Scholar]
- Phosphatidylinositol 3-kinase inhibition by copanlisib in relapsed or refractory indolent lymphoma. J Clin Oncol. 2017;35:3898-905.
- [CrossRef] [PubMed] [Google Scholar]
- The synthetic compound norcantharidin induced apoptosis in mantle cell lymphoma in vivo and in vitro through the PI3K-Akt-NF-κ B signaling pathway. Evid Based Complement Alternat Med. 2013;2013:461487.
- [CrossRef] [PubMed] [Google Scholar]
- Regulation of demethylation and re-expression of RASSF1A gene in hepatocellular carcinoma cell lines treated with NCTD in vitro. J Cancer Res Ther. 2015;11:818-22.
- [CrossRef] [PubMed] [Google Scholar]
- Small-molecule synthetic compound norcantharidin reverses multi-drug resistance by regulating sonic hedgehog signaling in human breast cancer cells. PLoS One. 2012;7:e37006.
- [CrossRef] [PubMed] [Google Scholar]
- Small-molecule multidrug resistance-associated protein 1 inhibitor reversan increases the therapeutic index of chemotherapy in mouse models of neuroblastoma. Cancer Res. 2009;69:6573-80.
- [CrossRef] [PubMed] [Google Scholar]
- Inhibition of multidrug resistance protein 1 (MRP1) improves chemotherapy drug response in primary and recurrent glioblastoma multiforme. Front Neurosci. 2015;9:218.
- [CrossRef] [PubMed] [Google Scholar]
- Quantitative high-throughput analysis of DNA methylation patterns by base-specific cleavage and mass spectrometry. Proc Natl Acad Sci U S A. 2005;102:15785.
- [CrossRef] [PubMed] [Google Scholar]
- Mass spectrometry to classify non-small-cell lung cancer patients for clinical outcome after treatment with epidermal growth factor receptor tyrosine kinase inhibitors: A multicohort cross-institutional study. J Natl Cancer Inst. 2007;99:838-46.
- [CrossRef] [PubMed] [Google Scholar]
- Serum proteomic study on EGFR-TKIs target treatment for patients with NSCLC. Onco Targets Ther. 2013;6:1481-91.
- [CrossRef] [PubMed] [Google Scholar]
Co-Corresponding Author:
Prof. Dr. Alexzander Asea, Founder, President and CEO, NampEVA BioTherapeutics LLC, Dover, DE - 19901, United States and Professor of Medicine and Director, Precision Therapeutics Proteogenomics Diagnostics Center, Department of Medicine, Division of Hematology/Oncology, Eleanor N. Dana Cancer Center, University of Toledo College of Medicine and Life Sciences, 3000 Arlington Avenue, Toledo, OH - 43614, United States. president@nampeva.com