Translate this page into:
Molecular genetics changes in gallbladder carcinoma
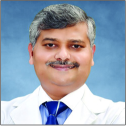
*Corresponding author: Dr. Puneet Kumar, Department of General Surgery, Institute of Medical Sciences, Banaras Hindu University, Varanasi, Uttar Pradesh - 221005, India. puneetimsbhu@gmail.com
-
Received: ,
Accepted: ,
How to cite this article: Verma K, Dixit R, Singh J, Tiwary SK, Khanna AK, Narayan G, et al. Molecular genetics changes in gallbladder carcinoma. Int J Mol Immuno Oncol 2020;5(2):49-61.
Abstract
Gallbladder carcinoma (GBC) is the fifth most common cancer of gastrointestinal tract with higher preponderance in women. This tumor is a highly aggressive disease with an overall 5-year survival of <5%. The disease presents in advanced stages where the treatment is ineffective. Different causative factors are documented to be linked with GBC pathogenesis such as environmental, microbial, metabolic, and molecular. One of the most important causing factors is molecular genetic alterations which are associated with GBC. Genetic mutations can be germline or somatic that involving proto-oncogenes, tumor suppressor genes, cell cycle, and growth factors. Several researches have been done to identify altered genes responsible for gallbladder carcinogenesis but the exact mechanism behind molecular pathogenesis still needs to be spread out. In this review, we summarized some of the important genetic changes reported in GBC to date that can be explored to achieve better understanding in gallbladder carcinogenesis.
Keywords
Gallbladder carcinoma
Oncogenes
Tumor suppressor genes
Cell cycle regulators
Adhesion molecules
INTRODUCTION
The first description of the gallbladder carcinoma (GBC) was given by Maxmillan de Stol in 1777. GBC is a very lethal and aggressive of the biliary cancers with a poor prognosis and the shortest median survival duration.[1-5] The symptoms and signs of GBC are vague and the clinical presentation is nonspecific may include abdominal pain, weight loss, fever, and jaundice, and any of these can be found in cholecystitis.[6-8] Most cancers originate in the fundus (fundus [60%], body [30%], or neck [10%]).[9] GBC has been reported to be 3–5 times more common in females than males.
The frequency of GBC progressively increases with age, even though it varies significantly in different parts of the world and also incidence varies within a country.[3,10-13] In India, the frequency rate of GBC is greatly higher in the North (e.g., incidence in Delhi is 4.5/100,000 for men and 10.1/100,000 for women) as compared with the South (e.g., in Chennai, Mumbai, Trivandrum, and Bengaluru incidence is 1.2/100,000 for men and 0.9/100,000 for women).[3,13] Histologically, the predominance of GBC is adenocarcinomas (80–95%),[14,15] and the leftover less common types include undifferentiated or anaplastic carcinoma (2–7%), squamous cell carcinoma (1–6%), and adenosquamous carcinoma (1–4%).[3,4,6,16-18]
The etiology of the disease is influenced by several risk factors which include exposure to certain chemicals and heavy metals, age, female gender, family history, obesity, cholelithiasis, chronic cholecystitis, porcelain gallbladder, polyps, and chronic infection by salmonella species along with various genetic factors.[3,14,15,19,20] Molecular genetic factors play an important role in gallbladder carcinogenesis.
GENETIC AND MOLECULAR CHANGE
GBC is a multi-factorial disease involving numerous genetic changes. In view of the present knowledge, genetic and molecular changes in GBC are still needs to be explored.[21-24] Some common aberrations include the activation of oncogenes, inactivation of tumor suppressor genes (TSGs), presence of microsatellite instability, and epigenetic changes mainly caused by atypical promoter methylation of gene areas are the various common factors reported till now. The serious of genetic changes leading to gallbladder cancer development is still not well known.[21,25] The present article will describe some of the important genetic changes reported in GBC [Table 1].
Genes | Major normal function | Molecular genetic changes in GBC | Expression in GBC | References | |
---|---|---|---|---|---|
Oncogene | K ras | GTPase activity and hydrolyzes guanosine triphosphate to guanosine diphosphate and are involved in signal transduction, molecular switches in the cell cycle | Point mutation | Higher (10–67%) | [24,31,40,47,93] |
EGFR | Active form of EGFR stimulates intracellular pathways that promote cell proliferation and angiogenesis and also to evasion of apoptosis | Point mutation and overexpression | Higher (63.4%) | [40,47,93] | |
HER2/neu (ERBB2) | HER2 is plasma membranebound cell surface receptor of the tyrosine kinase gene family and its protein clusters in cell membrane that play a role in tumorigenesis | Overexpression | Higher (16–64%) | [40,93] | |
Tumor suppressor gene | TP53 | TP53gene encodes a protein that binds to DNA, which in turn stimulates p21 that interacts with a cell cycle regulator (cdk2) | Overexpression, point, frameshift mutation | Higher (58.3–100%) | [40,93] |
FHIT | Encodes a cytoplasmic protein with diadenosine triphosphate (Ap3A) with hydrolase activity, and it involves in regulation of DNA replication, and apoptosis | Downregulation, MSI, and LOH | Lower (45–75%) | [21,40,93] | |
Retinoblastoma | Dysfunctional of Rb results in unscheduled cell proliferation, apoptosis, and widespread developmental defects, leading to embryonic death | Downregulation, MSI, and LOH | Lower (58.5%) | [40,93] | |
Adhesion molecules | MUC1 | Play a role in cell to cell interactions, signaling, and involvement in metastasis | Higher expression | Higher (78%) | [40,93] |
Erythrocyte CR1 | CR1 can act as a negative regulator involved in the complement cascade, which mediates immune adherence and phagocytosis through inhibiting both the classical and alternative pathways | Low activity | Lower | [40,93] | |
Cadherins | Tissue invasion (play an important role in cellcell adhesion) | Overexpression of Ncadherin, Pcadherin and lower expression of Ecadherin | Higher expression of Ncadherin (55%), Pcadherin (53%), and loss of Ecadherin expression (67%) | [21,93,94] | |
Angiogenesis factor | TSP1 | Involved in the regulation of cell growth, adhesion, proliferation, motility, cytoskeletal organization, inflammation and wound healing, and differentiation of various cell types | Higher expression | Higher (74.5%) | [93,96] |
COX2 | Catalyzed conversion of free arachidonic acid to prostaglandins and involved in the regulation of inflammation, cell growth, and tumor development | Overexpression | Higher (59.2–71.9%) | [73,93] | |
VEGFA | The VEGFA is a vigorous proangiogenic agent involved in the carcinogenesis | Overexpression | Higher (81%) | [93,104] | |
Cell cycle regulators | Cyclin E | Involved in the regulation of transition from the G1 phase to the S phase of cell cycle | Overexpression | Higher (33%) | [40,93] |
Cyclin D1 | Regulate the G1 phase of the cell cycle | Overexpression | Higher (41–68.3%) | [40,93] | |
p27Kip1 | It is an important cellcycle regulatory protein that interacts with cyclin CDK2 and CDK4, inhibiting cell cycle progression at G1. Its degradation is caused by its CDK dependent phosphorylation, and successive ubiquitination by SCF complexes is required for the cellular transition from quiescence to the proliferative state | Low expression | Lower (43–65%) | [93,113] | |
Apoptosis | Caspases | Involved in apoptosis and inflammation | Overexpression | Higher (77–95%) | [40,93] |
Bcl2 | Play an important role in neoplasia by inhibiting tumor cell apoptosis | Overexpression | Higher (34.7%) | [40,93] | |
Survivin | Survivin is a class of the IAP family which inhibit caspase activation and lead to negative regulation of apoptosis or programmed cell death | Overexpression | Higher (67.5%) | [124,125] |
GBC: Gallbladder carcinoma, EGFR: Epidermal growth factor receptor, FHIT: Fragile histidine triad, MSI: Microsatellite instability, LOH: Loss of heterozygosity, CR1: Complement receptor 1, TSP1: Thrombospondin-1, COX-2: Cyclooxygenase-2, VEGF-A: Vascular endothelial growth factor A, CDK: Cyclin-dependent kinase, IAP: Inhibitor of apoptosis
Oncogenes
Oncogenes cause alteration in normal cells to transform it into cancerous cells (with abnormal cell growth) is considered as oncogenes. Oncogenes are usually mutated forms of normal cellular genes (proto-oncogenes) that get altered in the gallbladder cancer signaling cascade. When a proto-oncogene altered, it becomes a “gain of function mutant gene” that can become permanently activated and influence cancer formation.[26]
K-ras
The ras gene family is a group of three closely associated cellular proto-oncogenes which play an significant role in the etiology of human cancers. There are three members of the ras family, namely N-ras, H-ras, and K-ras genes.[27] Many pathogenic mutations have been described in K-ras oncogene in GBC tissue.[21,28-30] K-ras encodes a 21-kD protein that is situated on the inner side of the plasma membranes that are a small GTP-binding cytoplasmic protein that has intrinsic GTPase activity and hydrolyzes guanosine triphosphate to guanosine diphosphate. K-ras protein that is bound to guanosine triphosphate is active, involved in signal transduction, and molecular switches in the cell cycle.[24,31,32] K-ras gene mutations identified in GBC generally affects codons 12, 13, and 61[21,33] results in inability to hydrolyze bound guanosine triphosphate and incapability to switch back to the inactive configuration, resultant inappropriate growth signal.[24] Most of the mutations were found in codon 12 of K-ras gene, and mutations of codons 13 and 61 were less common.[24,34-36] In North India, K-ras codon 13 mutation is more frequent (about one-third) than codon 12 and 61.[21,33] The identified rates of K-ras mutations were 5–57% for gallbladder cancers.[24,37-39]
GBC connected with an Anomalous Pancreaticobiliary Duct Junction have a high K-ras gene mutation rate in 50–83% of cases and this indicate that the detection of K-ras mutation is a valuable molecular diagnostic marker for GBC in this category of patients.[33,40,41] Although mutation of K-ras gene has never been identified in GBC having adenoma carcinoma sequence of development.[21,42]
Epidermal growth factor receptor (EGFR)
The EGF RTK family consists of four members: EGFR (ErbB1, and HER1), ErbB2 (HER2, neu in rodents), ErbB3 (HER3), and ErbB4 (HER4).[43-46] EGFR is a transmembrane receptor belonging to the ErbB family of receptor tyrosine kinases consists of an extracellular ligand-binding domain, a transmembrane domain, a short juxtamembrane section, a tyrosine kinase domain, and a tyrosine-containing C-terminal tail have a molecular weight of 170 kd.[47-49] The mature EGFR is made up of a single polypeptide chain of 1186 amino acid residues and a substantial amount of N-liked oligosaccharide.[50] There are six mammalian ligands that bind to EGFR, including itself EGF, transforming growth factor-α, amphiregulin, heparin-binding EGF-like growth factor, betacellulin, and epiregulin.[51]
Ligand binding to the EGFR receptor induces a conformational change of the receptor ectodomain that allows for receptor dimerization and autophosphorylation through tyrosine kinase activity of several tyrosine residues within the COOH-terminal tail of the receptors.[11,44,52-54] Autophosphorylation causes a chain of intracellular pathways that possibly result in cancer-cell proliferation, blocking apoptosis, activating invasion and metastasis, and stimulating tumor-induced neovascularization.[55]
EGFR is normally present within the plasma membrane of many types of normal cells, it is commonly over-expressed or over-activated in malignant cells. When active, it stimulates intracellular pathways that promote cell proliferation and angiogenesis, in addition to evasion of apoptosis.[47] Since the 1980s, a number of reports defined the overexpression of EGFR in a variety of epithelial tumors, supporting the hypothesis that dysregulation of EGFR gene expression and signaling could play a pivotal biological role in cancer.[56]
In 1995, Lee and Pirdas were the first to report a correlation between the membrane receptor EGFR and cases of GBC and other bile duct tumors, and its association with gallbladder dysplasia and chronic calculous cholecystitis.[48,57] EGFR overexpressed in gallbladder, common bile duct, and ampullary carcinomas but not in non-malignant situations of the gallbladder and biliary tract[58] such as in GBCs (63.4% and 70.7%) and dysplasia (71.4% and 85.7%) than in simple hyperplasia (15.4% and 27%) and normal gallbladder (0%).[40,59] The EGFR mutations, including activating and resistant mutations, frequently occur in exons 18–21 of the EGFR gene encoding the adenosine triphosphate-binding pocket of the intracellular TK domain.[56] Somatic mutations of the EGFR tyrosine kinase domain are reported in nearly 15% of the GBC.[58] Therapeutic targeting of EGFR/HER2 pathways increases the anti-proliferative effect of gemcitabine in GBC as shown by an earlier study, this can become a marker for poor prognosis.[21,60]
HER2/neu gene (ErbB2)
The HER2/neu gene is a proto-oncogene located on the 17q12-q21 chromosomal region and acts as an oncogene in several human cancers.[61,62] The role of HER family of tyrosine kinases playing an important role in the pathogenesis of cancer is well established. Among the four HER family proteins, HER2 has the strongest catalytic kinase activity and is the favored partner for dimerization with other HER family proteins.[63] The HER2/neu receptors undergo dimerization and transphosphorylation of their intracellular domains, which trans-activate numerous intracellular signaling molecules and adaptor proteins (Shc), which in turn lead to the activation of downstream pathways, such as ras-RAF-MEK ERK1/2 and PI3k-AKT-mTOR resulting in cancer cell proliferation and cell survival.[63,64] HER-2/neu (ERBB-2) overexpression reported to occur in 15.7%–63.6% cases of GBC. Overexpression of HER- 2/neu is important for carcinogenesis of gallbladder cancers and helpful for detection of early diagnosis.[40]
TSGS
TSGs are cellular genes that regulate cell division, DNA repair, and apoptosis (tell cells when to die/programmed cell death). When TSGs became non-functional, cells could get uncontrolled growth, leads to cancer. Allelic loss of TSGs, as well loss of heterozygosity (LOH) at polymorphic loci becomes the hallmarks of different cancers.[64]
TP53
TP53 is well-known TSG, situated on the short arm of chromosome 17p13,[31] encodes a 53-kD nuclear phosphoprotein which acts as a “Guardian of the genome” transcriptor factor, mainly in response to DNA damage by ultraviolet irradiation, γ-irradiation, and carcinogens[25,27,65,66] and has numerous mechanisms of anticancer function and plays an important role in maintenance of genome integrity, apoptosis, genomic stability, and inhibition of angiogenesis etc.[21,67]
TP53 gene is involved in cellular processes, such as gene expression, DNA repair, and apoptosis. Dysregulation in TP53 gene leads to cancer cell proliferation and immortality. Until the present, targeting TP53 gene mutations have evidenced to be a challenge. A number of approaches have been proposed: (1) Wee-1 kinase inhibitors together with DNA harmful agents, (2) inhibitors of the p53-MDM2 contact, which result in p53 stabilization, and (3) vascular endothelial growth factor (VEGF) receptor/VEGF-targeting agents in patients with TP53 mutations.[68] Mutations in TP53 gene are reported in a majority of human malignancies[69,70] and point mutations of the TP53 gene are among the greatest common genetic alterations in human cancers and are supposed to perform a critical role in the pathogenesis of many malignancies including GBC.[27,29,36-38,65,70-72] However, a missense mutation or a deletion of one p53 allele often consequences in the lack of the wild-type p53 protein, result in the loss of tumor suppressor function and permits the promotion of carcinogenesis.[70] Studies of exons 5–8 and exons 9 of the TP53 gene have been shown point mutations in 27%– 70% of GBCs.[21,27,38,40,73,74] Mutated p53 proteins have a longer half-life as compared to their wild-type p53 product.[25,63,67,75] TP53 abnormalities have been shown in patients of two regions, i.e., Japan and Chile with a high occurrence of GBCs.[70,73]
Fragile histidine triad (FHIT)
FHIT is a TSG that lost during gallbladder carcinogenesis, which reported on chromosome 3p. There are four distinct 3p regions (3p22-24, 3p21.3, 3p14.2, and 3p12) found in GBC with frequent LOH and have been reported by refined allele- type analysis.[31] A high incidence of LOH in the FHIT region was identified in both carcinoma and dysplastic epithelium of the gallbladder.[31,76] The loss of FHIT prompts global genome instability, mostly through nucleotide imbalance, spontaneous replication stress, and DNA breaks whereas methylation of the promoter, frameshift mutation, and elevated expression are common in GBC. In the gallbladder, FHIT allelic loss was detected in 13% of samples with chronic cholecystitis, 40% of dysplasia, and 76% of invasive carcinomas.[40,76-78]
Wistuba et al. reported the high correlation between immunostaining in the specimen and allelic loss.[74,76] and also noted that of a reduction in expression of FHIT in GBC.[74,78] Recent studies have reported that FHIT did not show any important tendency with respect to gallbladder cancer patient’s survival.[21]
Retinoblastoma (Rb) gene
Rb gene is tumor-suppressor gene encodes a protein (pRb) that acts by altering the activity of transcription factors in human Rb. Many types of cancer are mutated by Rb gene. Rb is a gene that has been found to be present in 100% of the patients with cholecystitis and adenoma, while it is deleted in carcinoma of the gallbladder in 18%–67% of the patients. It has been reported the expression of the pRb correlates with stage, grade, and overall survival.[79] In other study, Rb protein was found to be expressed in 24% of carcinoma, 32% of gallstones, and 50% of normal gallbladders.[80]
ADHESION MOLECULES
Cell adhesion molecules (CAMs) are proteins by nature, positioned on the cell surface involved in the association with other cells or with the extracellular matrix (ECM). Diverse CAMs (immunoglobulin such as protein CAM, cadherins, selectins, and integrins) take part in intercellular and cell-ECM interactions in case of cancer. Some adhesion molecules play a crucial role in the development of repeated, invasive, and distant metastatic cancer conditions.[81]
MUC1
Mucins are glycoproteins with high molecular weight. It provides a protective layer on epithelial surfaces, lubricating roles in the normal epithelium of gastrointestinal organs and significantly altered during neoplastic, paraneoplastic condition and are involved in cell-cell interactions, signaling, and metastasis.[40,82,83] Normal mucosal cells secrete a variety of different mucins such as MUC1, MUC2, MUC3, MUC4, MUC5A, and MUC6.[82,83] The MUC1 is located on chromosome 1q21 and MUC2, MUC5AC, and MUC6 on 11p15.5.[74,84] MUC1 is a transmembrane associated mucin large cell surface mucin glycoprotein expressed in a variety of cells in most glandular and ductal epithelial cells.[82] It has been reported that MUC staining on immunohistochemistry mainly in the cytoplasm and/or the cell membrane rather than the nucleus.[74,85,86] Chang et al. characterized the expression profile of MUC1, significantly higher than MUC5AC[40,87] and MUC6 in GBC. The expression of MUC1 is absent in normal gallbladder mucosa, whereas MUC5AC and MUC6 are much diffused.[40,88] MUC1 levels are significantly increased in gallbladder cancer, MUC2 and MUC5AC expression levels are reduced in gallbladder cancer when compared with dysplastic tissue as well carcinoma in situ, while the findings in the case of the two studies reporting expression levels of MUC6 are contradictory.[74,82,85-88] Finzi et al. have proposed that the MUC5AC is overproduced in gallstone disease by an inflammation-dependent EGFR cascade.[74,89] Previous studies have revealed that altered or an inappropriate mucin gene expression is related to tumor development, invasiveness, and prognosis.[87]
Erythrocyte complement receptor 1 (CR1)
Erythrocyte CR1 present on erythrocytes is a membrane protein which plays a significant role in immune clearance by bringing immune complexes from peripheral blood to macrophages in liver and spleen.[90] It has been reported that the rate of clearance of immune complexes from the circulation is directly proportional to the number of CR1 molecules expressed on erythrocytes.[90-92] CR1 can act as a negative controller of the complement cascade, mediates immune adherence and phagocytosis, and obstructs both the classic and alternative pathways.[40,91]
Erythrocytes from different healthy people may show up to 10-fold variation in the number of CR1 molecules per cell that may vary in the range of 50–1200 molecules per cell.[90] Several single nucleotide polymorphisms have been found in the CR1 gene, and minimum three polymorphisms of CR1 gene are associated with erythrocyte surface density of CR1 molecules, which in turn related to the rate of immune complex clearance from the circulation. The most widely studied three single nucleotide polymorphisms of CR1 gene are A3650G (His1208Arg), intron 27 HindIII (T520C), and Pro1827Arg.[90] The number and adhering activity of CR1 on erythrocyte in patients with GBC was reported to be lower as compared to patients with chronic cholecystitis, cholelithiasis, and healthy controls.[40,91]
E-cadherins (CDH1)
E-CDH1 is one of the most important molecules in cell-cell adhesion in epithelial tissues. It is localized on the surfaces of epithelial cells in regions of cell-cell contact known as adherens junctions. E-CDH1 is located on chromosome 16q22.1, which is critical for the development and Ca2+ maintenance of dependent cell adhesion, polarity, and differentiation of epithelial cells. It has been found that the significant differences in the protein expression of E-CDH1 between normal, inflamed, and cancerous gallbladder tissues. Reduced expression of E-CDH1 was reported in 67% of GBC. It has been observed that loss of E-CDH1 expression was a more prominent event in GBC as compared to chronic cholecystitis, xanthogranulomatous cholecystitis, and normal GB. Many reports suggested that the expression of E-CDH1 diminishes as the tumor progresses.[93,94]
ANGIOGENESIS FACTOR
In most cancerous condition, the development of malignant tumors is dependent on the genesis of new blood vessels (angiogenesis) to nourish malignant tumors.[95]
Thrombospondin-1 (TSP-1)
TSP-1 is a trimeric 450 kDa glycoprotein of the ECM and consists of three identical disulfide-bonded chains that affect cell adhesion, motility, and growth. TSP-1 has been reported in platelets and it is secreted by platelets, fibroblast cells, smooth muscle cells, monocytes, macrophages, and numerous neoplastic cells.[96-98] TSP has been involved in the regulation of cell growth, cell adhesion, proliferation, cell motility, cytoskeletal organization, inflammation and wound healing, and differentiation of different cell types.[96-99] TSP-1 is supposed to be a significant regulator of tumor growth and lymph node metastasis. TSP-1 expression was lacking in normal cells, even T1 cancers, but expressed 74.5% in T2 and T3 GBC along with high expression of lymph node metastasis.[40,96]
Cyclooxygenase-2 (COX-2)
COX enzyme is catalyzed conversion of free arachidonic acid to prostaglandins.[73,100,101] Isoform of COX, COX-2, is induced by mitogen, cytokines, and growth factors which involved in regulation inflammation, cell growth, and tumor development.[40,102] Over-expression of COX-2 was detected in GBC cases with high proportions in dysplasia and invasive carcinoma as compared with normal epithelium[74,100] COX-2 expression reported to be reduced in adenocarcinoma (59.2%) when compared with dysplastic epithelium (70.3%).[74,103] It has also been noted that the increased neovascularization induced by COX-2 might be responsible for the poor prognosis of human GBC patients.[73]
VEGF-A
The VEGF-A is a potent proangiogenic agent involved in many types of carcinogenesis and an attractive target for cancer therapy. It has been reported that high expression of VEGF-A was observed in 81% of GBC and 5.1% of chronic cholecystitis. VEGF-A is highly expressed in GBC and correlates with poor prognosis, suggesting that VEGF-A expression might be used as a biomarker for predicting malignant behavior and for identifying a subset of patients.[104,105]
CELL CYCLE REGULATORS
The cell cycle is regulated by several mechanisms that fortify correct cell division. Evading from normal cell cycle regulation is critical for the various processes of carcinogenesis. Cell cycle regulators more often altered in cancers are those governing G1-S-phase progression. G1-S transition is governed through interaction of various types of molecules, such as cyclin-dependent kinases (CDKs), their positive regulators, cyclins (D1, D2, D3, and E), and their negative regulators, CDK inhibitors, and Rb protein.[106] Very less information is available about the cell cycle regulators which get altered in GBC.[40]
Cyclin E
Cyclins are important proteins in cell-cycle regulation that is associated with and trigger different cyclin-dependent kinases at different stages of the cell cycle and allows them to pass through particular stage in the cycle.[107] Cyclin E is a regulatory subunit of the cell cycle kinase CDK2 and plays an important role in normal cell proliferation and development through promotion of S phase of the mammalian cell cycle.[108,109] Cyclin E seems to have the most significant role in many different types of tumor.[107,108] Cyclin E was expressed in the adenocarcinoma of gallbladder tissue in 33.3% of patients, whereas adenoma expressed 12.5%[40,98] which suggests that higher expression of cyclin E in adenocarcinoma of the gallbladder is related to a higher rate of cell proliferation.[107]
Cyclin D1
Restriction point present in cell-cycle progression, the restriction point of G1-S is very important. Cyclin D1 may promote the progression of the cell cycle to S by CDK4/ CDK6-mediated phosphorylation of the Rb protein, which is, in turn, regulated by CDK inhibitors, such as p16INK4 protein.[110] Cell-cycle drive is normally regulated by cyclins and cyclin inhibiting proteins.
Cyclin D1 plays role in cell cycle progression through two mechanisms: (a) Cyclin D1-CDK complexes deactivate Rb protein by phosphorylation; and (b) these complexes bind and sequester Cip/Kip proteins stoichiometrically.[106] Dysregulation of cyclin D1 plays a critical role in various malignancies. The previous study reported that the abnormal cyclin D1 expression rates in GBC and adenoma were significantly higher than those in chronic cholecystitis.[110] The expression rates of anomalous cyclin D1 were found in 68.3% and 57.1% GBC and gallbladder adenoma, respectively. These expression rates were significantly higher than those observed in chronic cholecystitis (7.1%)[40,110] specimens with overexpression of cyclin D1 showed a higher frequency of lymphatic permeation, venous permeation, and lymph node metastasis.[40,111]
Cyclin-dependent kinase inhibitor 1B (p27Kip1)
p27kip1 protein (p27), a cyclin-dependent kinase inhibitor, has an inhibitory effect on the G1-to-S phase transition in the cell cycle through its negative effects on cyclin E/CDK2 and cyclin A/CDK2. Lower expression of p27Kip1 is associated with poor prognosis in a variety of malignant diseases.[112,113] In the case of GBC, lower expression was reported in 43–65% cases.[93]
APOPTOSIS
Apoptosis was primarily described by its morphological characteristics, such as cell shrinkage, membrane blebbing, nuclear fragmentation, and chromatin condensation. Apoptosis is a gene-regulated program that has profound implications in developmental biology. Cell counts can be regulated by factors that affect cell survival as well as those that regulate proliferation and differentiation. Moreover, the reason for apoptosis implies that programmed cell death (PDC), i.e., apoptosis may be disrupted by a mutation in pro- apoptotic genes that show that defects in apoptotic genes are thought to be contributed to a number of human diseases, ranging from neurodegenerative disorders to malignancy.[114]
Caspases (CASP)
Studies have shown that the alteration in apoptotic pathway genes can cause defective apoptosis.[115] CASPs are very conserved intracellular aspartate-specific cysteine proteolytic enzymes, which are capable to cleave proteins with a consensus sequence at as tartaric acid alanine residues and play significant intermediaries of the apoptotic pathway.[116-118] Fourteen different CASPs have been reported in mammalian cells.[116]
CASP-8 is an important apoptosis regulatory gene which was assessed in cancer studies. They suggest that low penetrance variants in CASP-8 gene may affect GBC vulnerability.[115,116] Furthermore, many studies have been shown that active CASP-3 is required to induce apoptosis in response to chemotherapeutic treatments using treatments of taxanes, 5-fluorouracil, and doxorubicin.[119]
CASP-3 is a 32-kDa pro-enzyme, which was cleaved into 12 and 17-kDa subunits. Two 12-kDa and two 17-kDa subunits of the CASP-3 subunits were re-associated to form the functionally active CASP-3 enzyme. The initiator CASPs (CASP 8, 9, and 10) are activated by apoptotic stimulation factor, which further activates effector CASPs.[120] After activation of the effector CASP molecules which include CASP-3, 6, and 7 that initiates the cleavage of many important cellular proteins which include poly (ADP-ribose) polymerase, gelsolin, inhibitors of CASP-activated DNase, 4-GDI, α- and β-fodrin, and EGFRs.[118-120] CASPs caused blebbing of the membrane, nuclear destruction condensation of chromatin, and DNA fragmentations.[117-119] CASP-3 plays crucial role in apoptosis so that we evaluated the expression levels of procaspase-3 and activated CASP-3 in GB tumor tissue samples.[119] The level of apoptosis was higher in Grade II-III as compared with Grade I tumors or epithelial dysplasia, and also, tumors invading beyond serosa or into other organs (TI-T4) had a higher apoptotic index than other tumors (average apoptotic index was 0.68 ± 0.91%).[117] CASP 3 expression reported in 95% of GBC cases. CASP 6 and 8 expression was also observed, each in 77% GBCs.[40,117]
Bcl-2
Bcl-2 is an intracellular membrane protein capable of obstructing the PDC prompted by various stimuli. The Bcl- 2 gene is an anti-apoptotic membrane-associated molecule that exists in the nuclear envelope and outer membrane of mitochondria.[121] The Bcl-2 family proteins are significant regulatory components of the intracellular apoptotic pathway which is an important death regulator of mitochondrial integrity and mitochondria-regulated apoptosis.[121,122] Bcl-2 protein is supposed to play a role in cancer development by inhibiting tumor cell apoptosis.[122,123] Bcl-2 was reported over-expressed in 34.7% of the gallbladder cancer.[40,122]
Survivin
Survivin is the most effective member of human inhibitor of apoptosis. Survivin expressed in the G2/M phase of the cell cycle that plays a role in cell division, cell survival, and proliferation. Survivin overexpression is reported during fetal development but low in healthy adult tissues. Overexpression of survivin has been studied in various cancers. Survivin expression was significantly overexpressed in GBC than cholelithiasis. The results from different studies suggested its role in gallbladder carcinogenesis.[124,125]
OTHER GENES
Hedgehog pathway genes
Hedgehog signaling promotes tumor growth and it might take part in the proliferation of tumor stem cells.[79] The hedgehog pathway is an emerging therapeutic target for several cancers, including GBC. However, only one study has been reported so far.[126] Novel sonic hedgehog mutations were found 10 GBC patients.[126] Thus, further research is required to unravel the diagnostic role of hedgehog pathway genes.
Wnt signaling pathway genes
Yadav et al. reported genetic variants in Wnt signaling pathway which was found to be significantly associated with increased risk and poor survival. The study also depicted that AXIN-2 and APC play an important role in the regulation of the stability of β-catenin in the Wnt signaling pathway. Thus, the deregulation of β-catenin is an important event in the genesis of gallbladder carcinogenesis. Genetic variants of GLI-1, AXIN-2, and APC genes consistently showed association across all analyses, suggesting genetic variants are associated with advanced GBC susceptibility and treatment outcomes.[127]
Methylene tetrahydrofolate reductase (MTHFR) gene
The MTHFR gene is essential for DNA methylation and synthesis. Polymorphisms in the MTHFR gene have been studied as possible risk factors for a variety of diseases including cancer. A recent study concluded that increased frequency of the A1298C MTHFR gene polymorphism was higher in GBC in comparison to cholelithiasis and normal control and associated with gallbladder carcinogenesis.[128]
Non-coding RNAs (ncRNAs)
The transcriptome studies and genome tiling arrays specify that more than 90% of the human genome transcribes ncRNAs, while only 2% is thought to be transcribed and translated into proteins (Khandelwal et al., 2015). ncRNAs can be classified into two groups based on their size: (i) One group includes short RNAs <200 nucleotides (nt) in length, such as micro RNAs (generally between 22 and 25 nt), snoRNAs, and piwiRNAs (between 26 and 31 nt), and (ii) the other group includes long ncRNAs (lncRNAs) of around 200 nt or more.[129-131]
MicroRNAs (miRNAs)
miRNAs are small (18–25 nt), noncoding, and single- stranded RNA molecules that regulate gene expression at post-transcriptional and translational levels.[132] Nearly, 30% of the global gene expression is probably regulated by miRNAs. Aberrant expression of multiple miRNAs has been reported to be associated with many human cancers, and >50% of miRNA genes are found to be present with a satisfactory response to chemotherapy located in cancer- related chromosomal regions.[133] Numerous studies have reported aberrant miRNA expression in gallbladder cancer tissues and suggested specific miRNAs that are functionally involved in gallbladder cancer development.[134,135]
lncRNAs
lncRNAs, normally found as endogenous cellular RNAs, lack the Kozak consensus sequence and open reading frame of significant lengths. Although few studies have shown their ability to encode proteins that means these bi- functional RNAs can have both protein-coding and coding- independent functions.[130,136]
Initially thought to represent transcriptional noise in early RNA-Seq studies, lncRNAs are now regarded as functional RNA elements, generally expressed at low levels in a tissue- specific and time-restricted manner, with half-lives varying from <2 h to more than 16 h, and a median half-life of ~3.5 h. lncRNAs play significant roles in many biological processes, such as transcription, splicing, translation, protein localization, epigenetic gene expression, cell structure integrity, cell cycle, heat shock response, imprinting, stem cell pluripotency and reprogramming, embryogenesis, and regulation of the immune response. Because of their roles in the regulation of multiple molecular pathways associated with changes in gene expression, dysregulation of lncRNA associated with several types of cancer. Enforced expression of some cancer-promoting lncRNAs can enhance the proliferation and migration in cancer cells through regulating cell cycle protein and promoting epithelial–mesenchymal transition process. However, overexpression of some anticancer lncRNAs can inhibit the development of cancer, including proliferation inhibition, migration, suppression, and apoptosis induction.[129,137]
Some studies demonstrated that aberrantly expressed lncRNAs may serve a role in the occurrence and development of GBC. Although numerous lncRNAs have been discovered over the previous decade, the biological functions of lncRNAs in GBC and their underlying molecular mechanisms remain unclear.[138]
CONCLUSION
GBC is a highly lethal disease with wide geographical incidence. Despite the efforts of many investigators, GBC continues to represent a major challenge in oncology. Studies have shown variety of genes to be modified in GBC, but there is lack of sufficient knowledge about the exact role of these modifications in GBC. The present review article provided a summary of the molecular genetic changes occurred in GBC. This will be helpful for better understanding of gallbladder carcinogenesis. Further, exploration of complete genetic profile may provide a complete picture of the disease progression which will open new options for developing diagnostic biomarkers and targeted therapy in GBCs.
Declaration of patient consent
Patient’s consent not required as there are no patients in this study.
Financial support and sponsorship
Nil.
Conflicts of interest
There are no conflicts of interest.
References
- Diagnosis and management of gallbladder cancer. N Am J Med Sci. 2012;4:293-9.
- [CrossRef] [Google Scholar]
- Diagnostic and management issues in gallbladder carcinoma. Oncology (Williston Park). 1995;9:19-24.
- [Google Scholar]
- Carcinoma gallbladder. In: Recent Advances in Surgery. Vol 20. London: Churchill Livingstone; 1997. p. :69-87.
- [Google Scholar]
- Carcinoma of the gallbladder and extrahepatic bile ducts. Semin Oncol. 1988;15:106-15.
- [Google Scholar]
- Gallbladder carcinoma: Prognostic factors and therapeutic options. World J Gastroenterol. 2015;21:12211-7.
- [CrossRef] [PubMed] [Google Scholar]
- Gallbladder carcinoma update: Multimodality imaging evaluation, staging, and treatment options. AJR Am J Roentgenol. 2008;191:1440-7.
- [CrossRef] [PubMed] [Google Scholar]
- Diversity of complaints in manifesting carcinoma of the gallbladder. Ned Tijdschr Geneeskd. 2007;151:1083-6.
- [Google Scholar]
- Adjuvant radiotherapy in the treatment of gall bladder carcinoma: What is the current evidence. J Egypt Natl Canc Inst. 2016;28:1-6.
- [CrossRef] [PubMed] [Google Scholar]
- Gallbladder cancer worldwide: Geographical distribution and risk factors. Int J Cancer. 2006;118:1591-602.
- [CrossRef] [PubMed] [Google Scholar]
- Epidemiology and molecular pathology of gallbladder cancer. CA Cancer J Clin. 2001;51:349-64.
- [CrossRef] [PubMed] [Google Scholar]
- Annual Report of Population Based Cancer Registries of the National Cancer Registry Programme 1993 New Delhi: ICMR Publication; 1996. p. :18.
- [Google Scholar]
- Port site metastases in squamous cell carcinoma of the gallbladder. Isr Med Assoc J. 2014;16:177-9.
- [Google Scholar]
- Squamous cell carcinoma of the gallbladder associated with squamous metaplasia and adenocarcinoma in situ of the mucosal columnar epithelium. Acta Pathol Jpn. 1986;36:1879-86.
- [CrossRef] [PubMed] [Google Scholar]
- The WHO histological classification of tumors of the gallbladder and extrahepatic bile ducts. A commentary on the second edition. Cancer. 1992;70:410-4.
- [CrossRef] [Google Scholar]
- Carcinoma of the gallbladder. Histologic types, stage of disease, grade, and survival rates. Cancer. 1992;70:1493-7.
- [CrossRef] [Google Scholar]
- Gallbladder cancers: Associated conditions, histological types, prognosis, and prevention. Eur J Gastroenterol Hepatol. 2014;26:562-9.
- [CrossRef] [PubMed] [Google Scholar]
- Gallstone size and the risk of gallbladder cancer. JAMA. 1983;250:2323-6.
- [CrossRef] [PubMed] [Google Scholar]
- Frequency of gallbladder cancer in chile, a high-risk area. Int J Cancer. 1988;41:657-60.
- [CrossRef] [PubMed] [Google Scholar]
- Gallbladder cancer epidemiology, pathogenesis and molecular genetics: Recent update. World J Gastroenterol. 2017;23:3978-98.
- [CrossRef] [PubMed] [Google Scholar]
- Precancerous conditions of gallbladder carcinoma: Overview of histopathologic characteristics and molecular genetic findings. J Hepatobiliary Pancreat Surg. 2000;7:556-67.
- [CrossRef] [PubMed] [Google Scholar]
- Genome-wide allelotyping analysis reveals multiple sites of allelic loss in gallbladder carcinoma. Cancer Res. 2001;61:3795-800.
- [Google Scholar]
- Cellular and molecular biology of biliary tract cancers. Surg Oncol Clin N Am. 2002;11:995-1009.
- [CrossRef] [Google Scholar]
- Epithelial cell proliferation and gene mutation in the mucosa of gallbladder with pancreaticobiliary malunion and cancer. J Hepatobiliary Pancreat Surg. 1999;6:229-36.
- [CrossRef] [PubMed] [Google Scholar]
- Genetic abnormalities involved in the pathogenesis of gallbladder carcinoma. J Hepatobiliary Pancreat Surg. 1999;6:237-44.
- [CrossRef] [PubMed] [Google Scholar]
- K-ras gene mutation in gall bladder carcinomas and dysplasia. Gut. 1996;38:426-9.
- [CrossRef] [PubMed] [Google Scholar]
- APC, K-ras codon 12 mutations and p53 gene expression in carcinoma and adenoma of the gall-bladder suggest two genetic pathways in gall-bladder carcinogenesis. Pathol Int. 1996;46:333-40.
- [CrossRef] [PubMed] [Google Scholar]
- Relation between K-ras codon 12 mutation and p53 protein overexpression in gallbladder cancer and biliary ductal epithelia in patients with pancreaticobiliary maljunction. J Hepatobiliary Pancreat Surg. 2000;7:198-205.
- [CrossRef] [PubMed] [Google Scholar]
- Genetic alterations in gallbladder carcinoma. Surg Today. 2005;35:101-5.
- [CrossRef] [PubMed] [Google Scholar]
- Understanding ras: “It ain't over 'til it's over”. Trends Cell Biol. 2000;10:147-54.
- [CrossRef] [Google Scholar]
- Mutational spectrum of K-ras oncogene among Indian patients with gallbladder cancer. J Gastroenterol Hepatol. 2004;19:916-21.
- [CrossRef] [PubMed] [Google Scholar]
- High incidence of ras gene mutation in intrahepatic cholangiocarcinoma. Cancer. 1992;69:1115-8.
- [CrossRef] [PubMed] [Google Scholar]
- Cholangiocarcinomas in Japanese and Thai patients: Difference in etiology and incidence of point mutation of the c-ki-ras proto-oncogene. Mol Carcinog. 1992;6:266-9.
- [CrossRef] [PubMed] [Google Scholar]
- Mutations of p16Ink4/CDKN2 and p15Ink4B/MTS2 genes in biliary tract cancers. Cancer Res. 1995;55:2756-60.
- [Google Scholar]
- Allele-specific mutations involved in the pathogenesis of endemic gallbladder carcinoma in chile. Cancer Res. 1995;55:2511-5.
- [Google Scholar]
- K-ras and p53 mutations in stage I gallbladder carcinoma with an anomalous junction of the pancreaticobiliary duct. Cancer. 1996;77:452-8.
- [CrossRef] [Google Scholar]
- Multiple K-ras codon 12 mutations in cholangiocarcinomas demonstrated with a sensitive polymerase chain reaction technique. Cancer Res. 1991;51:3497-502.
- [Google Scholar]
- Genetic aberrations in gallbladder cancer. Surg Oncol. 2012;21:37-43.
- [CrossRef] [PubMed] [Google Scholar]
- K-ras, p53 mutations, and microsatellite instability (MSI) in gallbladder cancer. J Surg Oncol. 2006;93:644-9.
- [CrossRef] [PubMed] [Google Scholar]
- Genetic alterations in gallbladder carcinoma: A review. Nihon Geka Gakkai Zasshi. 1998;99:687-95.
- [Google Scholar]
- The epidermal growth factor receptor family: Biology driving targeted therapeutics. Cell Mol Life Sci. 2008;65:1566-84.
- [CrossRef] [PubMed] [Google Scholar]
- The epidermal growth factor receptor pathway: A model for targeted therapy. Clin Cancer Res. 2006;12:5268-72.
- [CrossRef] [PubMed] [Google Scholar]
- The role of epidermal growth factor receptor in cancer metastasis and microenvironment. Biomed Res Int. 2013;2013:546318.
- [CrossRef] [PubMed] [Google Scholar]
- A neu acquaintance for erbB3 and erbB4: A role for receptor heterodimerization in growth signaling. Cell. 1994;78:5-8.
- [CrossRef] [Google Scholar]
- Molecular genetics and targeted therapeutics in biliary tract carcinoma. World J Gastroenterol. 2016;22:1335-47.
- [CrossRef] [PubMed] [Google Scholar]
- Epidermal growth factor receptor immunoreactivity in gallbladder and extrahepatic biliary tract tumours. Pathol Res Pract. 1995;191:1087-91.
- [CrossRef] [Google Scholar]
- The epidermal growth factor receptor gene and its product. Nature. 1984;311:414-6.
- [CrossRef] [PubMed] [Google Scholar]
- Human epidermal growth factor receptor cDNA sequence and aberrant expression of the amplified gene in A431 epidermoid carcinoma cells. Nature. 1984;309:418-25.
- [CrossRef] [PubMed] [Google Scholar]
- Structure-function relationships for the EGF/TGF-alpha family of mitogens. Growth Factors. 1994;11:235-57.
- [CrossRef] [PubMed] [Google Scholar]
- Gallbladder cancer: Epidemiology and outcome. Clin Epidemiol. 2014;6:99-109.
- [CrossRef] [PubMed] [Google Scholar]
- Epidermal growth factor receptor expression in carcinoma gallbladder: A prospective study in Indian scenario. J Cancer Res Ther. 2016;12:959-62.
- [CrossRef] [PubMed] [Google Scholar]
- EGF-ERBB signalling: Towards the systems level. Nat Rev Mol Cell Biol. 2006;7:505-16.
- [CrossRef] [PubMed] [Google Scholar]
- ERBB receptors and cancer: The complexity of targeted inhibitors. Nat Rev Cancer. 2005;5:341-54.
- [CrossRef] [PubMed] [Google Scholar]
- Evaluation of P53, E-cadherin, cox-2, and EGFR protein immunoexpression on prognostic of resected gallbladder carcinoma. Arq Bras Cir Dig. 2014;27:126-32.
- [CrossRef] [PubMed] [Google Scholar]
- Somatic mutations of epidermal growth factor receptor in bile duct and gallbladder carcinoma. Clin Cancer Res. 2006;12:1680-5.
- [CrossRef] [PubMed] [Google Scholar]
- Significance of expression of epidermal growth factor (EGF) and its receptor (EGFR) in chronic cholecystitis and gallbladder carcinoma. Ai Zheng. 2003;22:262-5.
- [Google Scholar]
- Targeting EGFR/HER2 pathways enhances the antiproliferative effect of gemcitabine in biliary tract and gallbladder carcinomas. BMC Cancer. 2010;10:631.
- [CrossRef] [PubMed] [Google Scholar]
- HER2/neu-directed therapy for biliary tract cancer. J Hematol Oncol. 2015;8:58.
- [CrossRef] [PubMed] [Google Scholar]
- Overexpression of the HER2/neu gene: A new therapeutic possibility for patients with advanced gallbladder cancer. Gastrointest Cancer Res. 2014;7:42-8.
- [Google Scholar]
- HER2 as a therapeutic target in gallbladder cancer aye or nay? Transl Cancer Res. 2016;5:7-10.
- [Google Scholar]
- Tumour Suppressor Genes in Human Neoplasms Sudbury, MA, USA: Jones and Bartlett Publishers International; 1995.
- [Google Scholar]
- P53 and c-erbB-2 protein expression in adenocarcinomas and epithelial dysplasias of the gall bladder. J Pathol. 1993;170:67-72.
- [CrossRef] [PubMed] [Google Scholar]
- P53, the cellular gatekeeper for growth and division. Cell. 1997;88:323-31.
- [CrossRef] [Google Scholar]
- Involvement of DNA damage and repair in mutational spectra. Mutat Res. 2000;450:1-3.
- [CrossRef] [Google Scholar]
- Genomics of gallbladder cancer: The case for biomarker-driven clinical trial design. Cancer Metastasis Rev. 2016;35:263-75.
- [CrossRef] [PubMed] [Google Scholar]
- Molecular biology of gallbladder cancer: Potential clinical implications. N Am J Med Sci. 2012;4:435-41.
- [CrossRef] [PubMed] [Google Scholar]
- Alterations of p53 gene in gallbladder cancer patients of North India. Trop Gastroenterol. 2010;31:96-100.
- [Google Scholar]
- Mutations in the p53 tumor suppressor gene: Clues to cancer etiology and molecular pathogenesis. Cancer Res. 1994;54:4855-78.
- [Google Scholar]
- Aberrant expression of the p53 oncoprotein is a common feature of a wide spectrum of human malignancies. Oncogene. 1991;6:1699-703.
- [Google Scholar]
- Gallbladder cancer: A morphological and molecular update. Histopathology. 2009;55:218-29.
- [CrossRef] [PubMed] [Google Scholar]
- A genetic model for gallbladder carcinogenesis and its dissemination. Ann Oncol. 2014;25:1086-97.
- [CrossRef] [PubMed] [Google Scholar]
- Prognostic significance of HER-2 and p53 expression in gallbladder carcinoma in North Indian patients. Oncology. 2016;91:354-60.
- [CrossRef] [PubMed] [Google Scholar]
- Fragile histidine triad gene abnormalities in the pathogenesis of gallbladder carcinoma. Am J Pathol. 2002;160:2073-9.
- [CrossRef] [Google Scholar]
- Molecular Genetics of Gallbladder Cancer Chichester: John Wiley and Sons; 2015. p. :1-7.
- [Google Scholar]
- Expression of fhit, mlh1, and P53 protein in human gallbladder carcinoma. Cancer Lett. 2003;199:131-8.
- [CrossRef] [Google Scholar]
- Cyclin D1, retinoblastoma and p16 protein expression in carcinoma of the gallbladder. Asian Pac J Cancer Prev. 2013;14:2711-5.
- [CrossRef] [PubMed] [Google Scholar]
- The role of cell adhesion molecule in cancer progression and its application in cancer therapy. Acta Biochim Pol. 2004;51:445-57.
- [CrossRef] [PubMed] [Google Scholar]
- MUC 1 core protein as a marker of gallbladder malignancy. Eur J Surg Oncol. 2005;31:891-6.
- [CrossRef] [PubMed] [Google Scholar]
- Heterogeneity of mucin gene expression in normal and neoplastic tissues. Cancer Res. 1993;53:641-51.
- [Google Scholar]
- Mucin genes in gallstone disease. Clin Chim Acta. 2012;413:1466-71.
- [CrossRef] [PubMed] [Google Scholar]
- Expressive levels of MUC1 and MUC5AC and their clinicopathologic significances in the benign and malignant lesions of gallbladder. J Surg Oncol. 2012;105:97-103.
- [CrossRef] [PubMed] [Google Scholar]
- Immunohistochemical study of MUC1 and MUC5AC expression in gall bladder lesions. J Clin Diagn Res. 2017;11:EC12-6.
- [CrossRef] [PubMed] [Google Scholar]
- Phenotypic alterations of mucins and cytokeratins during gallbladder carcinogenesis. Pathol Int. 2004;54:576-84.
- [CrossRef] [PubMed] [Google Scholar]
- Expression of MUC2, MUC5AC and MUC6 apomucins in carcinoma, dysplasia and non-dysplastic epithelia of the gallbladder. Pathol Int. 1999;49:38-44.
- [CrossRef] [PubMed] [Google Scholar]
- MUC5AC, a gel-forming mucin accumulating in gallstone disease, is overproduced via an epidermal growth factor receptor pathway in the human gallbladder. Am J Pathol. 2006;169:2031-41.
- [CrossRef] [PubMed] [Google Scholar]
- Complement receptor 1 (A3650G rsaI and intron 27 hindIII) polymorphisms and risk of gallbladder cancer in North Indian population. Scand J Immunol. 2009;70:614-20.
- [CrossRef] [PubMed] [Google Scholar]
- Genomic determination of CR1 CD35 density polymorphism on erythrocytes of patients with gallbladder carcinoma. World J Gastroenterol. 2004;10:3480-4.
- [CrossRef] [PubMed] [Google Scholar]
- Role of E-cadherin gene in gall bladder cancer and its precursor lesions. Virchows Arch. 2010;456:507-14.
- [CrossRef] [PubMed] [Google Scholar]
- Gallbladder cancer in the 21st century. J Oncol. 2015;2015:967472.
- [CrossRef] [PubMed] [Google Scholar]
- Molecular identification of knops blood group polymorphisms found in long homologous region D of complement receptor 1. Blood. 2001;97:2879-85.
- [CrossRef] [PubMed] [Google Scholar]
- VEGF and the quest for tumour angiogenesis factors. Nat Rev Cancer. 2002;2:795-803.
- [CrossRef] [PubMed] [Google Scholar]
- Stromal expression of thrombospondin-1 is correlated with growth and metastasis of human gallbladder carcinoma. Int J Oncol. 1999;15:453-7.
- [CrossRef] [PubMed] [Google Scholar]
- Angiogenesis in cancer, vascular, rheumatoid and other disease. Nat Med. 1995;1:27-31.
- [CrossRef] [PubMed] [Google Scholar]
- Regulation of tumor growth and metastasis by thrombospondin-1. FASEB J. 1996;10:1183-91.
- [CrossRef] [PubMed] [Google Scholar]
- Platelet thrombospondin modulates endothelial cell adhesion, motility, and growth: A potential angiogenesis regulatory factor. J Cell Biol. 1990;111:765-72.
- [CrossRef] [PubMed] [Google Scholar]
- Expression of cyclooxygenase-2 is associated with p53 accumulation in premalignant and malignant gallbladder lesions. World J Gastroenterol. 2006;12:3425-9.
- [CrossRef] [PubMed] [Google Scholar]
- Cyclooxygenase-2 promotes angiogenesis by increasing vascular endothelial growth factor and predicts prognosis in gallbladder carcinoma. World J Gastroenterol. 2005;11:3724-8.
- [CrossRef] [PubMed] [Google Scholar]
- Expression of cyclooxygenase-2 in the subserosal layer correlates with postsurgical prognosis of pathological tumor stage 2 carcinoma of the gallbladder. Int J Cancer. 2002;98:427-34.
- [CrossRef] [PubMed] [Google Scholar]
- Cyclooxygenase-2 expression in the gallbladder of patients with anomalous arrangement of the pancreaticobiliary duct. J Pediatr Surg. 2003;38:585-9.
- [CrossRef] [PubMed] [Google Scholar]
- Immunohistochemical expression of vascular endothelial growth factor A in advanced gallbladder carcinoma. Appl Immunohistochem Mol Morphol. 2014;22:530-6.
- [CrossRef] [PubMed] [Google Scholar]
- Prognostic implications of estrogen receptor 1 and vascular endothelial growth factor A expression in primary gallbladder carcinoma. World J Gastroenterol. 2015;21:1243-50.
- [CrossRef] [PubMed] [Google Scholar]
- Cyclin D1 overexpression is a critical event in gallbladder carcinogenesis and independently predicts decreased survival for patients with gallbladder carcinoma. Clin Cancer Res. 2000;6:4272-7.
- [Google Scholar]
- Cyclin E expression in benign and malignant epithelial neoplasms of the gallbladder. Yonsei Med J. 1997;38:301-6.
- [CrossRef] [PubMed] [Google Scholar]
- Cyclin E overexpression and amplification in human tumours. J Pathol. 2003;200:375-82.
- [CrossRef] [PubMed] [Google Scholar]
- Correlation of aberrant expression of p53, rad50, and cyclin-E proteins with microsatellite instability in gallbladder adenocarcinomas. Genet Mol Res. 2009;8:1202-10.
- [CrossRef] [PubMed] [Google Scholar]
- Association of cyclin D1, p16 and retinoblastoma protein expressions with prognosis and metastasis of gallbladder carcinoma. World J Gastroenterol. 2005;11:744-7.
- [CrossRef] [PubMed] [Google Scholar]
- Nuclear cyclin D1 overexpression is a critical event associated with cell proliferation and invasive growth in gallbladder carcinogenesis. J Gastroenterol. 2000;35:142-9.
- [CrossRef] [PubMed] [Google Scholar]
- The role of p27kip1 protein expression on the biological behavior of intrahepatic cholangiocarcinoma. Hepatology. 2001;33:1118-23.
- [CrossRef] [PubMed] [Google Scholar]
- Low p27Kip1 expression is an independent prognostic factor in gallbladder carcinoma. Anticancer Res. 2003;23:675-9.
- [Google Scholar]
- Genetic landscape of gallbladder cancer: Global overview. Mutat Res. 2018;778:61-71.
- [CrossRef] [PubMed] [Google Scholar]
- Caspase-8 polymorphisms and risk of gallbladder cancer in a Northern Indian population. Mol Carcinog. 2010;49:684-92.
- [CrossRef] [PubMed] [Google Scholar]
- Apoptosis in gallbladder carcinomas and dysplasias, its relation to the expression of caspases 3, 6 and 8 and apoptosisregulating proteins bcl-2, mcl-1 and bax. Histol Histopathol. 2000;15:53-60.
- [Google Scholar]
- Inactivation of interleukin-1 beta converting enzyme by peptide (acyloxy)methyl ketones. Biochemistry. 1994;33:3934-40.
- [CrossRef] [PubMed] [Google Scholar]
- Expression of procaspase 3 and activated caspase 3 and its relevance in hormone-responsive gallbladder carcinoma chemotherapy. Korean J Intern Med. 2013;28:573-8.
- [CrossRef] [PubMed] [Google Scholar]
- Proliferation, cell cycle and apoptosis in cancer. Nature. 2001;411:342-8.
- [CrossRef] [PubMed] [Google Scholar]
- Prognostic value of bcl-2 expression in squamous cell carcinoma of the larynx: A systematic review. Int J Biol Markers. 2015;30:e155-60.
- [CrossRef] [PubMed] [Google Scholar]
- MiR-125b-5p enhances chemotherapy sensitivity to cisplatin by down-regulating bcl2 in gallbladder cancer. Sci Rep. 2017;7:43109.
- [CrossRef] [PubMed] [Google Scholar]
- Association of bcl-2 protein expression with gallbladder carcinoma differentiation and progression and its relation to apoptosis. Cancer. 1999;85:318-25.
- [CrossRef] [Google Scholar]
- Expression analysis of survivin and XIAP in gallbladder cancer: A case-control study in indo-gangetic plain. J Gastrointest Cancer. 2018;49:487-92.
- [CrossRef] [PubMed] [Google Scholar]
- Expression and clinicopathological significance of antiapoptotis protein survivin in gallbladder cancer. Indian J Pathol Microbiol. 2016;59:143-7.
- [CrossRef] [PubMed] [Google Scholar]
- Comparative analysis of mutational profile of sonic hedgehog gene in gallbladder cancer. Dig Dis Sci. 2017;62:708-14.
- [CrossRef] [PubMed] [Google Scholar]
- Association of WNT signaling pathway genetic variants in gallbladder cancer susceptibility and survival. Tumour Biol. 2016;37:8083-95.
- [CrossRef] [Google Scholar]
- Association of methylenetetrahydrafolate reductase gene polymorphism (MTHFR) in patients with gallbladder cancer. J Gastrointest Cancer. 2016;47:55-60.
- [CrossRef] [PubMed] [Google Scholar]
- Long non-coding RNA: A new paradigm for lung cancer. Mol Carcinog. 2015;54:1235-51.
- [CrossRef] [PubMed] [Google Scholar]
- Roles, functions, and mechanisms of long non-coding RNAs in cancer. Genomics Proteomics Bioinformatics. 2016;14:42-54.
- [CrossRef] [PubMed] [Google Scholar]
- The emerging role of long non-coding RNA in gallbladder cancer pathogenesis. Biochimie. 2017;132:152-60.
- [CrossRef] [PubMed] [Google Scholar]
- MicroRNAs: Genomics, biogenesis, mechanism, and function. Cell. 2004;116:281-97.
- [CrossRef] [Google Scholar]
- MicroRNAs and prostate cancer. Acta Biochim Biophys Sin (Shanghai). 2010;42:363-9.
- [CrossRef] [PubMed] [Google Scholar]
- MicroRNA expression and its implications for diagnosis and therapy of gallbladder cancer. Oncotarget. 2015;6:13914-21.
- [CrossRef] [PubMed] [Google Scholar]
- MicroRNA aberrations: An emerging field for gallbladder cancer management. World J Gastroenterol. 2016;22:1787-99.
- [CrossRef] [PubMed] [Google Scholar]
- Molecular mechanisms of long noncoding RNAs and their role in disease pathogenesis. Oncotarget. 2018;9:18648-63.
- [CrossRef] [PubMed] [Google Scholar]
- SPRY4-IT1: A novel oncogenic long non-coding RNA in human cancers. Tumour Biol. 2017;39:1010428317711406.
- [CrossRef] [PubMed] [Google Scholar]
- Long non-coding RNA expression profiles in gallbladder carcinoma identified using microarray analysis. Oncol Lett. 2017;13:3508-16.
- [CrossRef] [PubMed] [Google Scholar]