Translate this page into:
Clinical application of molecular genetics in acute myeloid leukemia
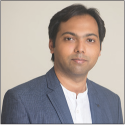
*Corresponding author: Naveen Gupta, Department of Clinical Hematology, Mahatma Gandhi Medical College and Hospital, Jaipur, Rajasthan, India. drnaveengupta1988@gmail.com
-
Received: ,
Accepted: ,
How to cite this article: Gupta A, Gupta N. Clinical application of molecular genetics in acute myeloid leukemia. Int J Mol Immuno Oncol 2022;3:102-7.
Abstract
With the increasing application of genetic testing, we have gradually understood the heterogeneous molecular nature of acute myeloid leukemia (AML). We have been able to identify a large number of translocations and single gene mutations to go with the previously known karyotypic abnormalities associated with this disease. This has led to a refinement in the way we diagnose, prognosticate, and treat this disease. The newer classification systems proposed by the World Health Organization and the International Consensus Classification systems show an increasing reliance on molecular genetics for the diagnosis and classification of AML. This has also generated situations where molecular genetics assume preference over morphologic assessment, namely, in the case of recurring genetic abnormalities where the standard 20% of blast count is not required, and in AML with underlying myelodysplasia where the disease shall be defined not by morphologic dysplasia but by the presence of certain cytogenetic and molecular abnormalities. The new prognostic system proposed by the European LeukemiaNet also places an emphasis on genetic mutations along with the previously known cytogenetic abnormalities. Similarly, the assessment of measurable residual disease (MRD) is recommended to be done by quantitative polymerase chain reaction (PCR) for core-binding factor and NPM1 mutated AML. In the future, next-generation sequencing is also expected to play a prominent role in the assessment of MRD alongside flow cytometry and PCR. Finally, precision oncology is supplementing conventional treatment regimens and patients with FLT3 and isocitrate dehydrogenase mutations are recommended to be offered the respective targeted agents. This review shall discuss the clinical implications of molecular genetics in the current management of AML.
Keywords
Acute myeloid leukemia
Genetics
Molecular classification
Measurable residual disease
Precision oncology
INTRODUCTION
Acute myeloid leukemia (AML) is one of the most common adult leukemias in adults and accounts for 1% of all cancer cases.[1] The disease is characterized by a proliferation of myeloid blasts but there is vast heterogeneity in the molecular signature of the malignant clone. For several decades, diagnosis of AML was dependent on morphology and immunophenotyping, risk stratification was largely determined by conventional karyotyping and treatment comprised of induction with “3+7” followed by consolidation with high dose cytarabine or allogeneic transplant. Molecular genetics has provided us with a better understanding of pathophysiology, refined risk stratification, and led to the development of novel targeted treatment modalities. The latest recommendations on diagnosis and treatment of AML reflect this changing paradigm and have been covered in this review.
EMERGING ROLE OF MOLECULAR MARKERS IN THE DIAGNOSIS OF AML
AML is a myeloid disorder characterized by genetic heterogeneity. Over the past few years, access to standardized molecular techniques has led to a better understanding of this disease. Next generation sequencing (NGS) has identified several important genetic mutations, grouping different patients of AML based on their mutated gene showing similar clinical profile, morphology, disease course, targeted therapy, and/or prognosis. Major scientific groups in hematology (World Health Organisation, International Consensus Classification and European LeukemiaNet) have proposed a new classification of AML mostly based on underlying genetic mutations with certain differences identified [Table 1].[2,3]
WHO | ELN | ICC | |
---|---|---|---|
Blast count | No cutoff limit for AML with defined genetic abnormality ≥20% blasts required for AML with CEBPA mutations and AML with BCR::ABL1 fusion | ≥10% blast count required for AML with a recurrent genetic abnormality ≥20% blasts required for AML with BCR::ABL1 fusion MDS/AML term added for 10–19% blasts in AML with TP53, AML-NOS and AML-MRC |
≥10% blast count required for AML with a recurrent genetic abnormality ≥20% blasts required for AML with BCR::ABL1 fusion MDS/AML term added for 10–19% blasts in AML with TP53, AML-NOS and AML-MRC |
AML with recurrent genetic abnormalities | t (9;11) fusions replaced by KMT2A rearrangements Two more rearrangements – MECOM and NUP98 Three fusions – DEK::NUP214, RBM15::MRTFA, and BCR::ABL1 NPM1 and CEBPA mutations added Myelodysplasia related gene mutations added |
Does not recognize rearrangements Seven fusions with recognized t (9;22)/BCR::ABL1 Somatic mutations with NPM1 and specific in-frame bZIP-mutated CEBPA |
Three rearrangements: KMT2A, RARA, and MECOM. 7 fusions Somatic mutations with NPM1 and specific in-frame bZIP-mutated CEBPA |
AML with mutated RUNX1 | Removed from the classification due to lack of specificity of a standalone type | Shifted to AML with myelodysplasia related gene mutations | Shifted to AML with myelodysplasia related gene mutations |
AML with mutated TP53 | Not recognized in AML classification | Recognized Monoallelic or biallelic TP53 mutations with VAF ≥10% is required |
Recognized Monoallelic or biallelic TP53 mutations with VAF ≥10% is required |
AML with myelodysplasia related gene mutations | Eight somatic mutations were identified: ASXL1, BCOR, EZH2, SF3B1, SRSF2, STAG2, U2AF1, or ZRSR2 | Nine somatic mutations identified: ASXL1, BCOR, EZH2, SF3B1, SRSF2, STAG2, U2AF1, or ZRSR2+RUNX1 | Nine somatic mutations identified: ASXL1, BCOR, EZH2, SF3B1, SRSF2, STAG2, U2AF1, or ZRSR2+RUNX1 |
The cutoff for blast percentage for the diagnosis of AML has always been a point of debate. As a rule of thumb, a blast count of ≥20% was established as an acceptable cutoff except for AML in the presence of defined or recurrent genetic abnormalities. No lower cutoff for blast count has been given by the WHO for this category; however, according to ELN and ICC, a minimum blast count of 10% is required. AML with BCR: ABL1 fusion and AML with CEBPA mutation still require at least 20% of blast count for diagnosis.[2,4]
AML with recurrent/defined genetic abnormalities has now been expanded with new genetic mutations such as BCR: ABL1 fusion, KMT2A rearrangement, DEK: NUP214 fusion, RBM15:MRTFA fusion, and MECOM rearrangement impacting the patient’s management and therapeutic decision. The WHO has replaced AML with t(9;11) fusions with KMT2A rearrangements because of several partner genes identified for KMT2A; however, ELN does not recognize these rearrangements and retains the fusions.[5]
TP53 mutations in AML are an entity representing a part of a spectrum of myeloid neoplasms with mutated TP53. The pathogenic TP53 mutation should have a variant allele frequency (VAF) of at least 10% with or without the loss of the wild allele.[5] The presence of complex cytogenetics with 17p deletion in the absence of TP53 mutation does not qualify for this entity. Clinically, these myeloid neoplasms have a very poor prognosis and are frequently associated with complex chromosomal abnormalities.[6,7] Diagnosis of AML with TP53 mutations takes precedence over AML with myelodysplasia-related gene mutations.[5]
A previously known entity of AML-MRC has been removed based on the recognition of more objective criteria of myelodysplasia-related gene mutations and myelodysplasia-related cytogenetic abnormalities. Detection of genetic mutations such as ASXL1, BCOR, EZH2, RUNX1, SF3B1, SRSF2, STAG2, U2AF1, and/or ZRSR2 in AML has led to the identification of a new AML category – “AML with myelodysplasia-related gene mutations” with poor prognosis. These genes have been commonly associated with AML arising from MDS or MDS/MPN.[8,9]
The list of mutations causing germline predisposition in AML is also expanding due to better molecular techniques. It is important to identify these mutations to identify other affected organs and also have an important therapeutic implication in matched sibling hematopoietic stem cell transplant.
Hierarchical classification of AML has been given by ICC where AML with recurrent genetic abnormalities takes precedence over all other categories. Among the remaining categories, AML with mutated TP53 supersedes AML with myelodysplasia-related gene mutations which supersedes AML with myelodysplasia-related cytogenetic abnormalities.
RISK STRATIFICATION
The ELN 2022 guidelines have made several changes in the risk stratification of AML at diagnosis.[5] A major change is that measuring the allelic ratio of FLT3-ITD mutations is no longer necessary. This change was made due to the lack of standardization of the assays for measuring the FLT3 allelic ratio. Furthermore, all FLT3-ITD-mutated AML now come under the intermediate risk category regardless of the coexistence of NPM1 mutations. This is because targeted treatment with FLT3 inhibitors has led to improved outcomes in all FLT3-mutated cases in the RATIFY trial.[10]
AML with myelodysplasia-related gene mutations is observed to carry a worse prognosis. In the 2017 ELN guidelines, the single gene mutations leading to inferior outcomes were TP53, RUNX1, and ASXL1. This list has now been expanded to include SRSF2, STAG2, BCOR, U2AF1, EZH2, SF3B1, and ZRSR2. The patients in ELN 2017 intermediate risk group were observed to carry a worse prognosis if carrying any of these myelodysplasia-related mutations and hence are now included in the adverse risk category.[11]
NPM1-mutated AML without FLT3-ITD carries a favorable outcome and those with FLT3-ITD fall under the intermediate risk group. A change in the recent risk stratification is that the presence of adverse risk cytogenetics overrides the significance of NPM1 mutation as these group of patients have been observed to have lower remission rates, inferior 5-year OS, and EFS with a higher 5-year cumulative incidence of relapse.[12]
With regards to CEBPA mutations, the previous understanding was that biallelic mutations carried a favorable prognosis and this was irrespective of the type of mutation seen. The understanding has now evolved as now it is observed that it is the in-frame mutations in the basic leucine zipper (bZIP) region of CEBPA that actually confer a favorable prognosis, regardless of whether they are monoallelic or biallelic.[13] CEBPA mutations in the transactivating domain or non-in-frame bZIP mutations do not confer this advantage.[14]
Cytogenetic risk groups in the adverse risk group have a few additions. t(3q26.2;v) involving rearrangements in the MECOM gene and t(8;16)(p11;p13) associated with KAT6A: CREBBP gene fusion are added to this group. Complex karyotype in AML is defined as having ≥3 unrelated chromosome abnormalities in the absence of other class-defining recurring genetic abnormalities. Patients with hyperdiploid karyotype in the absence of other adverse cytogenetics have been observed not to carry a worse outcome and these are now excluded from the definition of complex karyotype.[15] [Table 2] provides a side-by-side comparison of the 2017 and 2022 ELN risk stratification recommendations.[5,16]
Risk group | ELN 2017[15] | ELN 2022[4] |
---|---|---|
Favorable | t (8;21)(q22;q22.1); RUNX1-RUNX1T1 | t (8;21)(q22;q22.1)/RUNX1::RUNX1T1 |
inv (16)(p13.1q22) or t (16;16)(p13.1;q22); CBFB-MYH11 | inv (16)(p13.1q22) or t (16;16)(p13.1;q22)/CBFB::MYH11 | |
Mutated NPM1 without FLT3-ITD or with FLT3-ITD low | Mutated NPM1 without FLT3-ITD | |
Biallelic-mutated CEBPA | bZIP in-frame mutated CEBPA | |
Intermediate | Mutated NPM1 and FLT3-ITD high | Mutated NPM1 with FLT3-ITD |
Wild-type NPM1 without FLT3-ITD or with FLT3-ITD low (without adverse-risk genetic lesions) | Wild-type NPM1 with FLT3-ITD | |
t (9;11)(p21.3;q23.3); MLLT3-KMT2A | t (9;11)(p21.3;q23.3)/MLLT3::KMT2A | |
Cytogenetic abnormalities are not classified as favorable or adverse | Cytogenetic and/or molecular abnormalities not classified as favorable or adverse | |
Adverse | t (6;9)(p23;q34.1); DEK-NUP214 | t (6;9)(p23;q34.1)/DEK::NUP214 |
t (v; 11q23.3); KMT2A rearranged | t (v; 11q23.3)/KMT2A-rearranged | |
t (9;22)(q34.1;q11.2); BCR-ABL1 | t (9;22)(q34.1;q11.2)/BCR::ABL1 | |
inv (3)(q21.3q26.2) or t (3;3)(q21.3;q26.2); GATA2, MECOM (EVI1) | inv (3)(q21.3q26.2) or t (3;3)(q21.3;q26.2)/GATA2, MECOM (EVI1) | |
t (8;16)(p11;p13)/KAT6A::CREBBP | ||
t (3q26.2;v)/MECOM (EVI1)-rearranged | ||
-5 or del (5q); -7; -17/abn (17p) | -5 or del (5q); -7; -17/abn (17p) | |
Complex and monosomal karyotype | Complex and monosomal karyotype | |
Wild-type NPM1 and FLT3-ITD high | ||
Mutated RUNX1, ASXL1, and TP53 | Mutated ASXL1, BCOR, EZH2, RUNX1, SF3B1, SRSF2, STAG2, U2AF1, or ZRSR2, TP53 |
MEASURABLE RESIDUAL DISEASE (MRD)
Assessment of MRD after initial treatment is one of the most powerful tools for the determination of long-term prognosis and planning of further treatment. The 2021 consensus document on MRD measurement by the ELN sought to standardize the methodology and cutoffs for MRD in AML.[17] The measurement of MRD in AML may be done by flow cytometry or polymerase chain reaction (PCR) and the baseline immunophenotype and molecular profile of the disease determines the method to be employed.
Molecular MRD by PCR is the preferred method when there is a presence of a quantifiable AML-related mutation. This is employed for mutated NPM1; CBFB: MYH11, RUNX1:RUNX1T1, KMT2A: MLLT3, DEK: NUP214, BCR: ABL1 gene fusions; and WT1. The most robust data are available for NPM1 mutated and core-binding factor AML. The assessment is to be done from blood after two cycles of induction, from bone marrow at the end of treatment, and subsequently for follow-up-every 3 months from bone marrow or every 4–6 weeks from blood.[17] PCR-based MRD is considered negative if undetectable with Ct ≥40 in at least two of three replicates with at least ≥10,000 (preferably ≥30,000) copies of the housekeeping gene measured. Specifically for NPM1-mutated AML, there may be MRD persistence at low levels (<2%) without portending an unfavorable prognosis and this is labeled MRD-LL.
Flow cytometry-based MRD assessment is recommended for all cases without the abovementioned molecular. LAIP (leukemia-associated immunophenotype) and Dfn (different from normal immunophenotype) are the markers employed to identify the malignant clone. MRD by flow cytometry is considered negative if <0.1%.
NGS-based methods for MRD quantification are not recommended to be used as a standalone method at present. Their current utility is to be used as an adjunct to the abovementioned methods and for use as a research tool. Mutations associated with clonal hematopoiesis of indeterminate potential should not be used as MRD markers – these include DNMT3A, TET2, and ASXL1 also to be excluded from the analysis are germline mutations that may be present with a VAF>50%. Error corrected panel-based sequencing is to be employed.[18]
INDIVIDUALIZED TREATMENTS FOR SPECIFIC MOLECULAR TARGETS
A complete discussion on the treatment of AML is beyond the scope of this review. We shall briefly discuss the currently approved drugs targeting disease-specific mutations in AML.
FLT3 inhibitors
The recognition of FLT3-ITD mutations as a molecular marker for inferior prognosis in AML along with its high incidence (nearly 30%) prompted efforts to devise targeted treatments for this condition. This mutation leads to constitutive activation of FLT3 kinase resulting in increased proliferation and survival of myeloid blasts.[19] Several multikinase inhibitors have been tried in this setting but the most robust data are with midostaurin and it is approved for clinical use in the upfront setting. Giltertinib, a second generation FLT3 inhibitor has been approved in the relapsed refractory setting.
The clinical utility of midostaurin in newly diagnosed patients with FLT3-ITD-mutated AML was studied in the randomized, placebo, and controlled RATIFY trial. Patients were randomized to receive midostaurin 50 mg twice daily or a placebo from days 8 to 21 alongside induction and consolidation chemotherapy. This was followed by 12-month maintenance. An allogeneic transplant was also offered wherever feasible. Patients in the midostaurin arm had higher rates of complete remission (59% vs. 54%) and better 4-year survival (51.4% vs. 44.3%).[20] It must be noted that an allogeneic transplant was a major determinant of the survival benefit and a higher number of patients in the midostaurin arm underwent a transplant. Hence, midostaurin in initial therapy followed by an allogeneic transplant should be offered to FLT3-ITD-positive AML patients.[21] The benefit of midostaurin is also observed in the elderly population.[22] The role of maintenance midostaurin in this setting is still not clear and requires further studies.
Gilteritinib is an oral FLT3 inhibitor approved for use in relapsed refractory FLT3-ITD AML patients who had previously been treated with intensive chemotherapy. In the ADMIRAL trial, single agent Gilteritinib 120 mg per day was observed to be superior to chemotherapy in this setting with higher remission rates and median overall survival.[23]
Quizartinib is an oral FLT3 inhibitor that has been studied in a randomized, placebo, and controlled trial alongside intensive induction and consolidation chemotherapy in FLT3+AML. The final peer-reviewed publication is awaited but results presented in 2022 showed improved OS and RFS in the quizartinib arm.[24]
Isocitrate dehydrogenase (IDH) inhibitors
Mutations in IDH 1 and 2 are seen in 8% and 12% of cases of AML, respectively.[25] The consequence of these mutations is the formation of 2-hydroxyglutarate and consequent DNA hypermethylation. Inhibition of mutant IDH-1 and 2 genes by respective inhibitors ivosidenib and enasidenib leads to the resumption of myeloid differentiation. These drugs are now recommended in both upfront and relapsed/refractory settings.
Ivosidenib is an orally active selective IDH-1 inhibitor. In a randomized and Phase 3 trial, ivosidenib (500 mg once a day) versus placebo along with azacytidine were used in patients of IDH-1-mutated AML unfit for standard chemotherapy. The ivosidenib arm had significantly superior OS and EFS.[26] This combination of azacitidine and ivosidenib is approved for elderly frail patients who are not candidates for intensive induction. It may also be used as a single agent in salvage settings for very frail patients. Enasidenib is an orally active IDH2 inhibitor that is approved for use in relapsed/refractory cases at a dose of 100 mg once daily.
CONCLUSION
The management of AML is slowly pivoting in the direction defined by molecular genetics. With the increasing availability of PCR-based assays and next generation sequencing techniques, we can refine the way we diagnose, prognosticate, and treat this disease. A lot of new insights have been gained regarding the pathophysiology of AML and many more changes in diagnostic and prognostic criteria are expected in the future. The heterogeneous nature of the disease means that there is a wide range of mutations that may become amenable to targeted therapy in the future.
Declaration of patient consent
Patient’s consent not required as there are no patients in this study.
Financial support and sponsorship
Nil.
Conflicts of interest
There are no conflicts of interest.
References
- Acute Myeloid Leukemia Cancer Stat Facts. SEER. Available from: https://seer.cancer.gov/statfacts/html/amyl.html [Last accessed on 2022 Sep 15]
- [Google Scholar]
- The 5th edition of the world health organization classification of haematolymphoid tumours. Myeloid and histiocytic/dendritic neoplasms In: Leukemia. Vol 36. 2022. p. :1703-19.
- [CrossRef] [PubMed] [Google Scholar]
- International consensus classification of myeloid neoplasms and acute leukemias: Integrating morphologic, clinical, and genomic data. Blood. 2022;140:1200-28.
- [CrossRef] [PubMed] [Google Scholar]
- Overexpression of wild-type or mutants forms of CEBPA alter normal human hematopoiesis. Leukemia. 2012;26:1537-46.
- [CrossRef] [PubMed] [Google Scholar]
- Diagnosis and management of AML in adults: 2022 recommendations from an international expert panel on behalf of the ELN. Blood. 2022;140:1345-77.
- [CrossRef] [PubMed] [Google Scholar]
- TP53 alterations in acute myeloid leukemia with complex karyotype correlate with specific copy number alterations, monosomal karyotype, and dismal outcome. Blood. 2012;119:2114-21.
- [CrossRef] [PubMed] [Google Scholar]
- TP53 mutation in patients with high-risk acute myeloid leukaemia treated with allogeneic haematopoietic stem cell transplantation. Br J Haematol. 2016;172:914-22.
- [CrossRef] [PubMed] [Google Scholar]
- Distinct Mutation Landscapes Between Acute Myeloid Leukemia With Myelodysplasia-Related Changes and De novo Acute Myeloid Leukemia. Am J Clin Pathol. 2022;157:691-700.
- [CrossRef] [PubMed] [Google Scholar]
- Acute myeloid leukemia ontogeny is defined by distinct somatic mutations. Blood. 2015;125:1367-76.
- [CrossRef] [PubMed] [Google Scholar]
- Impact of NPM1/FLT3-ITD genotypes defined by the 2017 European LeukemiaNet in patients with acute myeloid leukemia. Blood. 2020;135:371-80.
- [CrossRef] [PubMed] [Google Scholar]
- Added prognostic value of secondary AML-like gene mutations in ELN intermediate-risk older AML: ALFA-1200 study results. Blood Adv. 2020;4:1942-9.
- [CrossRef] [PubMed] [Google Scholar]
- Chromosomal abnormalities and prognosis in NPM1-mutated acute myeloid leukemia: A pooled analysis of individual patient data from nine international cohorts. J Clin Oncol. 2019;37:2632-42.
- [CrossRef] [PubMed] [Google Scholar]
- CEBPA-bZip mutations are associated with favorable prognosis in de novo AML: A report from the Children's Oncology Group. Blood. 2021;138:1137-47.
- [CrossRef] [PubMed] [Google Scholar]
- CEBPA mutations in 4708 patients with acute myeloid leukemia: Differential impact of bZIP and TAD mutations on outcome. Blood. 2022;139:87-103.
- [CrossRef] [PubMed] [Google Scholar]
- Hyperdiploidy with 49-65 chromosomes represents a heterogeneous cytogenetic subgroup of acute myeloid leukemia with differential outcome. Leukemia. 2014;28:321-8.
- [CrossRef] [PubMed] [Google Scholar]
- Diagnosis and management of AML in adults: 2017 ELN recommendations from an international expert panel. Blood. 2017;129:424-47.
- [CrossRef] [PubMed] [Google Scholar]
- 2021 Update on MRD in acute myeloid leukemia: A consensus document from the European LeukemiaNet MRD Working Party. Blood. 2021;138:2753-67.
- [CrossRef] [PubMed] [Google Scholar]
- Next-generation sequencing for measurable residual disease detection in acute myeloid leukaemia. Br J Haematol. 2020;188:77-85.
- [CrossRef] [PubMed] [Google Scholar]
- Targeting FLT3 mutations in AML: Review of current knowledge and evidence. Leukemia. 2019;33:299-312.
- [CrossRef] [PubMed] [Google Scholar]
- Midostaurin plus chemotherapy for acute myeloid leukemia with a FLT3 mutation. N Engl J Med. 2017;377:454-64.
- [CrossRef] [PubMed] [Google Scholar]
- Midostaurin reduces relapse in FLT3-mutant acute myeloid leukemia: The alliance CALGB 10603/RATIFY trial. Leukemia. 2021;35:2539-51.
- [CrossRef] [PubMed] [Google Scholar]
- Midostaurin added to chemotherapy and continued single-agent maintenance therapy in acute myeloid leukemia with FLT3-ITD. Blood. 2019;133:840-51.
- [CrossRef] [PubMed] [Google Scholar]
- Gilteritinib or chemotherapy for relapsed or refractory FLT3-mutated AML. N Engl J Med. 2019;381:1728-40.
- [CrossRef] [PubMed] [Google Scholar]
- AML-029 Quizartinib prolonged overall survival (OS) vs placebo plus intensive induction and consolidation therapy followed by single-agent continuation in patients aged 18-75 years with newly diagnosed FLT3-internal tandem duplication positive (FLT3-ITD+) acute myeloid leukemia (AML) Clin Lymphoma Myeloma Leuk. 2022;22(Suppl 2):S208-9.
- [CrossRef] [Google Scholar]
- Acute myeloid leukemia with IDH1 and IDH2 mutations: 2021 treatment algorithm. Blood Cancer J. 2021;11:107.
- [CrossRef] [PubMed] [Google Scholar]
- Ivosidenib and azacitidine in IDH1-mutated acute myeloid leukemia. N Engl J Med. 2022;386:1519-31.
- [CrossRef] [PubMed] [Google Scholar]