Translate this page into:
Understanding immunotherapy and CAR-T-cells: A narrative review
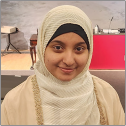
*Corresponding author: Mohammadi Maahtaab, School of Medicine, Royal College of Surgeons in Ireland, Dublin, Ireland. mohammadimaahtaa21@rcsi.com
-
Received: ,
Accepted: ,
How to cite this article: Maahtaab M. Understanding immunotherapy and CAR-T-cells: A narrative review. Int J Mol Immuno Oncol. 2024;9:7-11. doi: 10.25259/IJMIO_22_2023
Abstract
This comprehensive narrative review aims to delve into the various aspects of immunotherapy, with a special focus on the role of chimeric antigen receptor T (CAR-T) cells in modern medical interventions. To strengthen the understanding of the foundations of immunotherapy, a detailed exposition of the mechanisms underpinning the normal human immune response has been provided, encompassing both innate and adaptive immunity, along with the pivotal role of immune cells in neoplastic surveillance and immune editing. Furthermore, the review elucidates various active and passive immunotherapeutic interventions, providing a thorough overview of various agents and their mechanisms of action. It also delves into the structure of CAR-T-cells, their signaling cascades, and the means to fine-tune their effector functions. In addition, the review briefly throws light on the diverse clinical applications of CAR-T-cells that extend beyond its infamous applications in oncology to diseases with high healthcare burdens, such as autoimmune disorders, HIV, and cardiac fibrosis. This holistic exploration of the current state of immunotherapy and the dynamic landscape of CAR-T-cell therapy thus underscores the promise of these advancements in shaping a patient-centric approach to disease management and treatment.
Keywords
Immunotherapy
Chimeric antigen receptor T-cells
Chimeric antigen receptor molecules
Cancer
Tumor
INTRODUCTION – AN OVERVIEW OF THE IMMUNE SYSTEM
Understanding the mechanisms utilized by our immune cells to elicit inflammatory reactions is essential in understanding immunotherapy. The human immune response can broadly be divided into innate and adaptive immunity.
Innate immunity rapidly responds to pathogen associated molecular patterns and damage-associated molecular patterns (DAMPs) through pattern recognition receptors (PRRs) such as toll-like receptors (TLR) and cellular DNA sensors. Immune cells activated include neutrophils, eosinophils, natural killer (NK) cells, basophils, mast cells, dendritic cells, and macrophages. Antigen-presenting cells (APCs), such as dendritic cells and macrophages, activate adaptive immunity by presenting antigens on major histocompatibility complexes (MHCs) class 1 and 2.
Adaptive immunity’s first line involves T-cells, including CD4+ T helper cells and CD8+ cytotoxic T-cells (CTLs). CD8+ T-cells target viral and tumor antigens on MHC class 1, inducing cell death. CD4+ cells bind to pathogenic antigens on MHC class 2, which activate B-cells. Regulatory T-cells (TREGs) release anti-inflammatory cytokines and dampen the immune response through the activation of co-stimulatory surface molecules like CD28.[1,2]
B-cells have B-cell receptors (BCR) and, when activated, produce immunoglobulins (antibodies). Immunoglobulins cause neutralization of pathogens, activate the complement cascade and induce antibody-dependent cellular cytotoxicity (ADCC).
In neoplasms, immune cells undergo immunosurveillance and immunoediting in three stages: elimination (where immune cells detect and kill neoplastic cells), equilibrium (rate of immune cell induced neoplastic cell death equals the rate of proliferation), and escape phases. Escape mechanisms involve poor antigenicity, anti-apoptotic gene expression, anti-inflammatory cytokines, TREG activation, and checkpoint inhibitors, suppressing immune reactions.[3]
IMMUNOTHERAPY – THE STATE-OF-THE-ART
Immunotherapy is a novel biological therapy that exploits the aforementioned immunological mechanisms to amplify the immune system to target specific diseases. Compared to other forms of therapeutic interventions, immunotherapy is advantageous due to its targeted nature, ubiquitous involvement of the immune system in disease, and the ability to boost or suppress the immune system depending on the type of condition being treated.[4,5]
Classification of immunotherapeutic interventions
Active immunotherapy
Active immunotherapy is the direct stimulation of immune cells by the immunotherapeutic agent, mimicking the body’s immune response and producing memory cells.[6]
Immunogenic cell death inducers
Immunogenic cell death inducers are characterized by chemotherapeutics that are used to kill neoplastic cells, leading to the release of DAMPs. These DAMPs stimulate APCs, which then, activate downstream adaptive immune response through CD8+ T-cells, ultimately amplifying the tumor apoptosis or “immunogenic cell death.”[7,8]
PRR agonist
Several PRR agonists for transmembrane TLRs, which are expressed in most immune cells and help sense danger, have been developed to initiate a downstream signaling cascade that can effectively kill tumor cells.[7-9]
Dendritic cell activation
Administration of exogenous peptides or DNA-based antigens can lead to in vivo activation and differentiation of dendritic cells as APCs. Specifically, exogenous tumor-associated antigens (TAAs) can activate the dendritic cells, leading to the activation of tumor-specific Th cells or CTLs, ultimately killing the tumor cells. This mechanism has been used in the treatment of autoimmune disorders such as type-1 diabetes (T1D); by administering insulin, the TREG immunoinhibitory pathway is activated, thus reducing disease progression in T1D.[10]
Immunostimulatory cytokines
Exogenous cytokines stimulate innate immune cells such as macrophages and NK cells, as well as the maturation and differentiation of CD4+ and CD8+ T-cells, ultimately leading to inflammatory and apoptotic effects. For instance, interleukin-2 (IL-2) is used in the treatment of melanoma. Injection of anti-inflammatory cytokines, in theory, can also enable resolution in chronic conditions.
Therapeutic cancer vaccines (TCVs)
TCVs are based on in vitro transfection of dendritic cells to express TAAs. These dendritic cells extracted from the donor body are transfected (using RNA vectors or CRISPR-aided genetic modification), allowed to express TAAs, increase, and then transfused into the donor’s circulation, thus stimulating an immune response against tumor cells, and acting as a therapeutic rather than a preventive vaccine.
Checkpoint inhibitors
Immune cells often express coinhibitory molecules that elicit immunosuppressive effects. An example of these molecules include cytotoxic T lymphocyte-associated protein 4 (CTLA-4) and programmed cell death one on activated T-cells. The use of monoclonal antibodies to block these receptors is effective in re-activating the anti-tumor immune effects. An example of a Monoclonal Antibody (MAB) in clinical practice is ipilimumab, that blocks the aforementioned CTLA-4 receptor and is FDA approved for treatment of melanoma.[7,9]
Passive immunotherapy
Passive immunotherapy refers to the administration of immunological agents that indirectly engage the host immune system.
Anti-tumor monoclonal antibodies
Tumor-specific monoclonal antibodies bind and elicit antibody-based reactions. Naked antibodies elicit an immune response through complement activation, induction of apoptosis, and ADCC through NK cells, while conjugated antibodies are usually conjugated with active factors such as radioisotopes or toxins, where the antibody is used as a drug-delivery/targeting tool to achieve targeted therapy.
Oncolytic virus
Oncolytic viruses are either natural or genetically engineered that host themselves in specific tumor cells, replicate, and lead to tumor cell apoptosis while also triggering cytokine release, thus amplifying the immune response.
Tumor-infiltrating lymphocytes (TIL) therapy
TIL includes T-cells, B-cells, and NK cells, and TIL therapy focuses on boosting their immune response. In this process, tumor cells are biopsied, TILs are isolated and expanded using IL-2 and reinjected into the body.[7]
CAR-T-CELLS
CAR-T-cells are a form of passive immunotherapy characterized by T-cells that are genetically engineered to express receptors that can bind to specific ligands on target cells. The receptors are chimeric because they bind to a specific antigen while also inducing T-cell functions, which can then mediate cytotoxic effects. The evolution of CAR-T-cell therapy, especially in the field of oncology, has successfully aided in streamlining tumor cell death and regression in a targeted manner. The first-generation CART-cells involved CARs with an extracellular single-chain variable fragment (ScFv) and an intracellular CD3ζ. The present fourth-generation “Armored” CAR-T-cells consist of multiple co-stimulatory domains in the CAR as well as other protein molecules.[10,11]
Mechanism of CAR-T-cell production
T-cells are extracted through Leukapheresis from the host blood to avoid allogeneic rejection or graft-versus-host disease and are activated in vitro using immunostimulatory cytokines such as IL-2 and artificial APCs.
CARs are then introduced either through retroviral or lentiviral vectors or by gene editing using CRISPR-Cas9 and transcription activator-like effector nucleases.
CAR-T-cells are then grown on a large scale and are reintroduced into the host in single or multiple doses.[12]
General structure of CAR molecules
CAR molecules generically consist of two extracellular domains, a transmembrane domain, and two intracellular domains as seen in Figure 1.[13]

- Structure of second-generation chimeric antigen receptor. (CAR), ICOS: Inducible Costimulator.
The distal antigen-recognizing extracellular domain consists of a variable light chain and variable heavy chain derived from ScFv designed for a specific target-cell ligand.
The hinge domain links the distal extracellular domain to the transmembrane region and allows flexibility in forming immune synapses between the CARs and the target-cell ligands, usually derived from CD9 or CD28.
The transmembrane domain, derived from CD8 and CD28, regulates signaling.
The intracellular co-stimulatory domain consists of CD28 or 4-IBB domains or multiple domains.
The distal intracellular cytoplasmic domain, similar to the T-cell receptors (TCR) intracellular domain, consists of CD3ζ with 3 immunoreceptor tyrosine-based activation motifs (ITAMs) that aid downstream signaling.[10]
Signaling cascades and mechanism of action
On binding to the target cell ligand, the CARs become clustered and immobilized.
The transmembrane domain dimerizes or trimerizes, causing phosphorylation of the ITAM domain on CD3ζ.
This induces a signaling cascade involving tyrosine kinase ζ-associated protein of 70 kDa (ZAP70), leading to genetic transcriptional and translational upregulation of a variety of genes.
As a result of the signaling cascade, the CAR-T-cells proliferate, release cytokines, and cause necrosis through granzymes and perforins.
Downstream activation of various death ligands, BH3-interacting domain death agonists, and FAS-associated death domain protein binds to death receptors on tumors and initiates apoptosis.
Repeated stimulation of the CAR-T-cells post-binding leads to activation-induced cell death.
Fine-tuning CAR-T effector cells
The CAR-T-cells’ proliferative capacity, persistence, potency, and resistance to exhaustion can determine their efficiency in mediating cytotoxic effects. These parameters can be altered at a genetic level as well as by altering the individual domains on the CARs.[10]
Deletion of specific genes such as suppressor of cytokine signaling 1, RAS GTPase-activating protein 2, and CBLB (encoding an E3 ubiquitin ligase) increases cytotoxicity and proliferative capacity.
CAR gene integration with TRAC promoter aids CAR receptor internalization and re-expression on tumor relapse, creating “memory” CAR-T-cells.
Variations in the hinge domain length and ITAM functional domains impact CAR binding efficacy, downstream signaling, and resistance to cell death.
The co-stimulatory molecules, depending on whether it is CD28 or 4-IBB, can affect initial expansion, durability, persistence, and the CAR-T-cell fate.[11]
FURTHER CLINICAL APPLICATIONS OF CART-CELLS
Autoimmune disorders
Autoimmune disorders result from a loss of self-tolerance, leading to B-cells producing autoantibodies and suppressing regulatory T-cell mechanisms. TCR can be modified into chimeric autoantibody receptors targeting BCR on plasma B-cells, creating immunological blockage. Another approach involves switching CAR-T-cell phenotype to CAR-TREG through FOXP3 gene transduction, down-regulating T lymphocyte activation.[12]
HIV
CAR-T-cells with CD4+ domains can target the gp120 region of the HIV envelope, suppressing viral infection. Next-generation anti-HIV CARs with broadly neutralizing antibodies bind to gp120 and gp41, eliciting antiviral activity against infected cells.
Cardiac fibrosis
CAR-T-cells targeting fibroblast activation protein in activated fibroblasts show promise in curing fibrosis in animal studies. Intravenously introduced modified mRNA with targeted CD5 lipid nanoparticles generates Anti-fibroblast CAR-T-cells in vivo, treating cardiac fibrosis while minimizing toxic effects.[14]
CONCLUSION
Advances in immunotherapeutic interventions have aided in delivering patient-centered care by reducing the number of comorbidities compared to other invasive and aggressive interventions in chronic diseases such as cancer. The plethora of research in understanding the CAR-T-cells’ mechanism of action has further extended its applications to several diseases and can potentially overcome the existing limitations of CAR-T therapy, such as cytokine release syndrome and neurotoxicity. Strategies such as fine-tuning CAR-T effector cells, combination therapies, and enhancing safety profiles through genetic modifications are pivotal in overcoming these challenges.
Ethical approval
The Institutional Review Board approval is not required.
Declaration of patient consent
Patient’s consent was not required as there are no patients in this study.
Conflicts of interest
There are no conflicts of interest.
Use of artificial intelligence (AI)-assisted technology for manuscript preparation
The authors confirm that there was no use of artificial intelligence (AI)-assisted technology for assisting in the writing or editing of the manuscript and no images were manipulated using AI.
Financial support and sponsorship
Nil.
References
- Concise clinical immunology for healthcare professionals. United Kingdom: Routledge and CRC Press; Available from: https://www.routledge.com/concise-clinical-immunology-for-healthcareprofessionals/keogan-wallace-oleary/p/book/9780415298308 [Last accessed on 2023 Nov 20]
- [Google Scholar]
- Immuno-oncology: Understanding the function and dysfunction of the immune system in cancer. Ann Oncol. 2012;23(Suppl 8):viii, 6-9
- [CrossRef] [PubMed] [Google Scholar]
- Delivery technologies for cancer immunotherapy. Nat Rev Drug Discov. 2019;18:175-96.
- [CrossRef] [PubMed] [Google Scholar]
- Cancer immunotherapy: Pros, cons and beyond. Biomed Pharmacother. 2020;124:109821.
- [CrossRef] [PubMed] [Google Scholar]
- Types of biological therapy-SEER training. Available from: https://training.seer.cancer.gov/treatment/biotherapy/types.html [Last accessed on 2023 Nov 20]
- [Google Scholar]
- Classification of current anticancer immunotherapies. Oncotarget. 2014;5:12472-508.
- [CrossRef] [PubMed] [Google Scholar]
- Detection of immunogenic cell death and its relevance for cancer therapy. Cell Death Dis. 2020;11:1013.
- [CrossRef] [PubMed] [Google Scholar]
- Immunotherapy: Past, present and future. Nat Med. 2023;9:269-77.
- [CrossRef] [PubMed] [Google Scholar]
- Antigen-based immunotherapy for autoimmune disease: Current status. Immunotargets Ther. 2014;4:1-11.
- [CrossRef] [PubMed] [Google Scholar]
- Recent advances and discoveries in the mechanisms and functions of CAR T cells. Nat Rev Cancer. 2021;21:145-61.
- [CrossRef] [PubMed] [Google Scholar]
- Application of CAR-T cell therapy beyond oncology: Autoimmune diseases and viral infections. Biomedicines. 2021;9:59.
- [CrossRef] [PubMed] [Google Scholar]
- Co-stimulatory receptor signaling in car-T cells. 2022 Available from: https://www.mdpi.com/2218-273X/12/9/1303 [Last accessed on 2023 Oct 29]
- [CrossRef] [PubMed] [Google Scholar]
- The use of targeted LNP/mRNA technology to generate functional, transient CAR T cells and treat cardiac injury in vivo. J Cardiovasc Aging. 2022;2:23.
- [CrossRef] [Google Scholar]