Translate this page into:
Endocrine toxicity of cancer immunotherapy
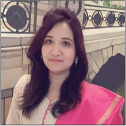
*Corresponding author: Silima Subhasnigdha Tarenia, Department of Endocrinology, Calcutta Medical College, Kolkata, West Bengal, India. drsilimatarenia@gmail.com
-
Received: ,
Accepted: ,
How to cite this article: Das N, Tarenia SS, Kalra S. Endocrine toxicity of cancer immunotherapy. Int J Mol Immuno Oncol. doi: 10.25259/IJMIO_19_2024
Abstract
Immune checkpoint inhibitors (ICPIs) based on the efficacious anti-tumour immune response become an integral part of a number of cancer therapies. However, their widespread use has been linked to an increase in adverse events affecting almost every organ system. Common ICPIs include cytotoxic T lymphocyte-associated protein 4 (CTLA-4) inhibitors, programmed death 1 (PD-1) inhibitors, and PD ligand 1 inhibitors. Among the ICPIs induced immune-related adverse events, endocrine events are particularly common. Thyroid gland is the most common endocrine organ affected by ICPIs, followed by the pituitary gland. Hypophysitis is specifically linked to anti-CTLA-4 therapy, while thyroid dysfunction is associated with anti-PD-1 therapy. Rare endocrine adverse effects such as diabetes mellitus and primary adrenal insufficiency can also occur with ICPI therapy and, if not promptly addressed, may be life-threatening. Combination therapy with anti-CTLA-4 and anti-PD-1 inhibitors results in the highest incidence of endocrinopathies. Although most ICPI-related endocrine dysfunction emerges within 12 weeks of starting the therapy, some may develop months to years later. While some endocrine issues may resolve spontaneously, central adrenal insufficiency and primary hypothyroidism tend to persist. Management of ICPI-induced endocrine dysfunction primarily involves hormone replacement and control of symptoms. This article aims to review the endocrine complications of ICPI therapy and offer guidance on screening and managing patients experiencing endocrine dysfunction in daily clinical practice.
Keywords
Immune checkpoint inhibitors
Endocrine dysfunction
Immune-related endocrine events
INTRODUCTION
Immune checkpoint inhibitors (ICPIs) based on the efficacious anti-tumor immune response become an integral part of a number of cancer therapies. The use of ICPIs has been linked to an increase in adverse events affecting almost every organ system.[1] Common ICPIs include cytotoxic T lymphocyte-associated protein 4 (CTLA-4) inhibitors such as ipilimumab and tremelimumab), programmed death 1 (PD-1) inhibitors such as nivolumab, cemiplimab, and pembrolizumab, and programmed death ligand 1 (PD-L1) inhibitors such as avelumab, atezolizumab, and durvalumab. CTLA-4 inhibits the proliferation and activation of T-cells by competing with CD28 for binding to CD80 and CD86.[2,3] PD-1, present on immune cells, binds with its ligand PDL1, presents on tumor cells macrophages, and induces immune tolerance.[4,5] Activation of PD1-PDL1 inhibits the activation of T-cells, promotes apoptosis of tumor-infiltrating lymphocytes, reduces inflammatory cytokines, and increases the anti-inflammatory interleukin 10, thus allowing tumor cells to escape immune surveillance.[5]
Non-specific T-cell activation induced by ICPIs can lead to side effects affecting up to 40–50% of treated patients.[6-8] Common immune-related adverse events (irAEs) include skin reactions, diarrhea, colitis, and hepatitis. Immune-related endocrine events (free) occurring in 8.1% of all cases are among the most common irAEs.[9-11] Thyroid gland is the most commonly affected endocrine organ, followed by pituitary, diabetes mellitus (DM), and primary adrenal insufficiency (PAI).[12-14] Combination of anti-CTLA-4 and anti-PD-1 therapies results in a higher occurrence of thyroid dysfunction and hypophysitis compared to using either treatment alone patients with irEEs which have better clinical response and better survival.[15]
It is suggested that immune activation induced by ICPIs destroys nearly all hormone producing cells, although there is a lack of histological evidence to confirm this hypothesis.[11] This condition often begins with a lazy inflammatory phase that goes unnoticed until significant cell destruction occurs. Thus, unlike non-endocrine toxicities, irEEs are frequently irreversible and do not resolve with glucocorticoid therapy.
THYROID
Thyroid dysfunction is the most prevalent endocrine disorder following ICPI therapy, ranging from overt hypothyroidism to hyperthyroidism. It affects 5% of patients treated with CTLA-4 inhibitors, 10% of patients treated with PD-1 or PD-L1 inhibitor monotherapy, and up to 15–20% on combination therapy.[11] Dysfunction typically occurs within weeks of ICPI initiation, with a median onset occurring between 18 and 123 days.[16-19] It initially presents as painless thyroiditis, progressing from a transient hyperthyroid phase to a euthyroid or hypothyroid phase. Furthermore, some patients initially present with subclinical or clinical hypothyroidism. Symptoms are usually mild and non-specific, often identified during regular monitoring, or may manifest with symptoms such as fatigue, depression, weight gain, constipation, and mental status alteration in severe cases.[20-22] Myxoedema coma, although reported, is rare.[23] Those presenting in the toxic phase of thyroiditis might exhibit symptoms such as weight loss, palpitations, anxiety, and tremors.[20-22] However, thyroid storm is rare.[24,25] Some patients have elevated autoantibodies, such as anti-thyroid peroxidase and anti-thyroglobulin.[19,26] High levels of these antibodies before treatment seem to be associated with ICPI-induced thyroid dysfunction.[27] Risk factors of ICPI-induced thyroid dysfunction include high baseline body mass index, baseline thyroid-stimulating hormone (TSH) levels, and prior use of tyrosine kinase inhibitors.[28] ICPI-induced Graves’ disease is much less common; however, it should be differentiated from the thyrotoxic phase of thyroiditis by measuring the TSH receptor antibody titer or an uptake scan. Also, the presence of Thyroid eye disease favors Graves’ disease. Graves’ disease is managed by anti-thyroid drugs.[29,30]
TSH level is the most sensitive and preferred test for diagnosing thyroid dysfunction in patients treated with ICPI. Primary hypothyroidism is indicated by TSH elevation with low free thyroxine (FT4) whereas central hypothyroidism secondary to pituitary disorder is diagnosed by low FT4 along with low normal to suppressed TSH. In case of thyrotoxicosis, low TSH is seen with elevated FT4 and triiodothyronine (T3). Thyroid function should be evaluated every 4–8 weeks or more frequently if indicated clinically in all patients treated with ICPI.[31,32] In hypothyroidism, if TSH is elevated above 10 mIU/mL on two separate occasions or mildly elevated along with low FT4 level without going for further investigations thyroid hormone should be replaced. Subclinical hypothyroidism is characterized by elevated TSH below 10 mIU/mL and a normal FT4 level. In such cases, treatment decisions should be personalized according to symptoms, age, and comorbidities.[33]
Hypothyroidism is treated with levothyroxine, with the dose adjusted based on age comorbidities, with a lower starting dose for advanced age. After initiating treatment, TSH levels should be checked 6–8 weeks later. For most patients with primary hypothyroidism, the dose of levothyroxine dose should be adjusted to achieve a normal TSH level. However, in central hypothyroidism, monitoring of FT4 is recommended instead of TSH, and cortisol levels should be checked and replaced if found low before initiating thyroid hormone to prevent adrenal crisis precipitation. Hyperthyroidism due to thyroiditis is managed symptomatically by beta-blockers and steroids in selected cases with close monitoring for progression to hypothyroidism, while hyperthyroidism secondary to Graves’ disease is treated with anti-thyroid drugs such as carbimazole, methimazole, surgery, or radioactive iodine based on clinical presentation and patient’s preference.
HYPOPHYSITIS
Hypophysitis, the inflammation of the pituitary gland is often resulting from infections, infiltrations, tumors, or autoimmune processes. ICPI-induced hypophysitis is most frequently triggered by CTLA-4 Inhibitor ipilimumab, with an incidence rate ranging from 1.8% to 17%[34] followed by anti-PD1/PD-L1 therapy at 0.5–1%.[35,36] Combination therapy with anti-CTLA-4 and anti-PD-1 increases the risk of hypophysitis compared to using anti-CTLA-4 therapy alone.[6] No specific risk factors have been identified for ICPI-induced hypophysitis, although some studies suggest potential risk factors such as age, male gender,[35] anti-pituitary antibodies, and certain human leukocyte antigen (HLA) alleles.[37] The higher incidence in male may be due to the frequent use of ICPI therapy in melanoma, which is more common in men. Symptoms usually present between 9 and 12 weeks after starting anti-CTLA-4 therapy and around 26 weeks for anti-PD-1/L1 therapy.[35] Symptoms are often non-specific, including headache and fatigue,[35,38] while signs from mass effects like visual defects are rare. Other symptoms include dizziness, poor appetite, nausea, weight loss, and decreased libido. The primary consequence of ICPI-induced hypophysitis is one or more pituitary hormone deficiencies, most commonly being central hypothyroidism, central adrenal insufficiency, and hypogonadotropic hypogonadism. In central hypothyroidism, low or low normal FT4 levels with low or inappropriately normal TSH levels are seen.[39] This condition is usually transient, and the thyroid axis recovers spontaneously in most of the cases. It is also important to note that non-thyroidal illness syndrome (NTIS) can present with similar biochemical thyroid function test abnormality; however, in NTIS, serum T3 decline is progressive, and T4 and TSH also decrease if the underlying illness does not improve. Central adrenal insufficiency, which is characterized by low cortisol and low adrenocorticotropic hormone (ACTH) levels, presents with hypotension and hyponatremia and sometimes can be life-threatening.[40] Cosyntropin (ACTH) stimulation test helps to diagnose adrenal insufficiency, although it may not rule out recent-onset central adrenal insufficiency and concurrent corticosteroid therapy. Central adrenal insufficiency is often permanent. Hypogonadotropic hypogonadism is characterized by low or inappropriately normal follicle-stimulating hormone and luteinizing hormone levels in the setting of low serum testosterone and low estradiol levels in men and women, respectively. Hypogonadotropic hypogonadism is usually transient and recovers spontaneously; however, it is difficult to distinguish it from functional hypogonadism secondary to underlying illness. Growth hormone deficiency[41] and abnormal prolactin levels are rare. The posterior pituitary is usually spared, and diabetes insipidus has rarely been reported in the literature.[42,43] Magnetic resonance imaging (MRI) often shows an enlargement of the pituitary, which may precede clinical diagnosis and usually resolves within weeks to months.[35] Mild-to-moderate pituitary enlargement with homogeneous or heterogeneous contrast enhancement with or without stalk thickening is usually seen on imaging.[44] However, a normal imaging does not rule out hypophysitis.[45] Treatment includes glucocorticoid replacement for adrenal insufficiency most commonly with hydrocortisone or prednisone with patient education about sick day dosing to prevent a crisis. For central hypothyroidism, glucocorticoids to be given before initiating thyroid hormone to avoid adrenal crisis.[46] Although monitoring in central hypothyroidism is based on FT4 levels, with a goal of keeping the value in middle half of reference range, TSH can also be monitored because an increase in TSH level after the diagnosis of central hypothyroidism may indicate recovery of the thyrotrophs. Higher doses of glucocorticoid therapy (prednisone 1–2 mg/kg followed by tapering dose) are indicated in patients with severe compressive symptoms such as headache and vision change.[32,47] Testosterone replacement is considered in men with hypogonadotropic hypogonadism and estrogen therapy in women based on individual risk assessment. Desmopressin is used for diabetes insipidus if severe dehydration and hypernatremia occur.[48] Severe dehydration and hypernatremia are classified as grade 4 toxicity, requiring temporary suspension of therapy.[47,49,50] High-dose glucocorticoids are usually given for severe hyponatremia.
PAI
The development of PAI induced by ICPI (ICPI-PAI) is rare.[51,52] However, combination therapy with PD-1 and CTLA-4 inhibitors carries a higher risk of PAI compared to monotherapy using PD-1 inhibitors.[53] Men and older adults are more susceptible to ICPI-PAI. Symptoms in patients with PAI include fatigue, nausea, vomiting, poor appetite, weight loss, nausea, hypotension, or adrenal crisis secondary to mineralocorticoid deficiency.[51,53,54] Diagnosed is confirmed by low morning cortisol levels along with an elevated ACTH level. Common findings include hyponatremia, metabolic acidosis, hyperkalemia (due to mineralocorticoid deficiency)[54] with hypoglycemia, and hypercalcemia being less frequent. Plasma renin is usually elevated; however, it should be interpreted correctly, especially if IV fluids are administered, as they can affect renin and aldosterone levels.[20] Patients with PAI should be initiated on glucocorticoid replacement. The dosage and method of administration (oral/parenteral) should be determined based on the clinical status of the patient. In acute phases, treatment is started with an initial bolus parenteral dose of 100 mg of hydrocortisone, followed by 50 mg every 6 hours, and then gradually tapered as the patient stabilizes. Long-term treatment involves lifelong glucocorticoid replacement therapy, typically 15–25 mg of hydrocortisone in divided doses or 5 mg prednisolone once daily. Mineralocorticoid replacement is also needed to prevent hypotension and hyperkalemia, with an initial fludrocortisone dose is 50–100 ug daily orally, adjusted based on clinical response and electrolytes.[55] ICPIPAI should be differentiated from adrenal insufficiency due to adrenal metastasis or adrenal hemorrhage by adrenal imaging. ICPI-induced adrenalitis typically shows bilaterally enlarged adrenals with smooth borders in contrast to metastasis, which presents with a nodular appearance with a distorted adrenal margin.[21]
DIABETES
DM has been observed in 0.2–1.4% of patients receiving ICPI therapy.[56,57] Studies indicate that ICPI-induced DM occurs in approximately 71% of individuals treated with anti-PD-L1 or anti-PD-1 monotherapy, 15% with combination therapy involving PD-1/PD-L1 and CTLA-4 blockade, and 3% with anti-CTLA-4 monotherapy.[11,57] ICPI-DM arises from autoimmune destruction of pancreatic b-cells, which express PD-L1.[11,58] Patients with ICPI-DM who tested positive for islet autoantibodies typically develop diabetes shortly after beginning therapy in contrast to those without these autoantibodies.[58] In addition, HLA DR4 has been strongly associated with the development of ICPI-DM.[59] Pancreatic inflammation induced by ICPI therapy can also lead to exocrine. The median age at diagnosis is 63 years. The onset of diabetes has been observed after a single dose or up to 17 doses and within a time frame ranging from 1 week to 12 months.[60-63]
ICPI-related DM is marked by acute onset of hyperglycemia, rapid progression to endogenous insulin deficiency, and a high risk of developing diabetic ketoacidosis (DKA) if not detected and treated with insulin. DKA symptoms include polyuria, polydipsia, nausea, vomiting, pain abdomen, and coma, while hyperglycemia may also be detected through blood tests. As hyperglycemia develops suddenly, hemoglobin A1c levels might appear normal. This type of DM results in complete endogenous insulin deficiency accompanied by low or undetectable C-peptide levels. Pancreatic imaging using ultrasound, computed tomography (CT), or MRI in certain studies has either been normal or indicated atrophy, enlargement, or signs of widespread inflammation.[64-66] Screening for ICPI-DM includes monitoring random blood glucose levels during each treatment cycle. Approximately 60–85% of ICPI-DM cases present with DKA.[67] Patients with DKA should promptly undergo treatment according to DKA protocol. This includes continuous intravenous insulin infusions, fluid resuscitation, and correction of electrolyte imbalances.[68] Lifelong insulin therapy is necessary for these patients, and their management should parallel that of classic type 1 DM.
HYPOPARATHYROIDISM
ICPI-induced hypoparathyroidism is an exceedingly uncommon endocrine complication.[69,70] Patients exhibit mild hypocalcemia and hyperphosphatemia with symptoms such as perioral tingling, numbness, muscle cramp, spasms, and tetany. Diagnostic evaluation should involve assessing serum calcium, albumin, and phosphorus.[71] On confirming hypocalcemia, measuring parathyroid hormone (PTH) levels can confirm hypoparathyroidism which is characterized by low or inappropriately normal PTH levels. Treatment typically involves calcium supplementation along with calcitriol administration to maintain normal serum calcium levels.
SCREENING RECOMMENDATIONS
Patients, family members, and physicians should receive thorough education regarding potential endocrine complications of ICPIs before starting treatment and continuously throughout the process. This education should be provided by a team comprising of endocrinologist and an oncologist. Individuals should be able to recognize and promptly report various symptoms or signs, such as severe weakness, unusual headache patterns, excessive sweating, palpitation, mood changes, constipation, diarrhea, polyuria, polydipsia, polyphagia, low appetite, nausea, vomiting, and abdominal pain.[47] Baseline assessment should include TSH, FT4, morning cortisol, glucose, and electrolyte levels. These tests should be repeated every 4–6 weeks interval during treatment, with another round of testing 4–6 weeks after the final cycle of immunotherapy to detect late-onset complications. [Table 1] outlines the appropriate clinical and laboratory evaluation for patients receiving ICPIs for potential endocrinopathies.
At baseline | Clinical evaluation for symptoms such as severe weakness, vision changes headache palpitation, weight loss or weight gain, diarrhea or constipation, mood changes, polyuria, polydipsia, polyphagia, low appetite, nausea, vomiting, pain abdomen Laboratory evaluation for TSH, FT4, morning cortisol, plasma glucose, and serum electrolytes |
Every 4–6 weeks and 4–6 weeks after the last treatment cycle | Clinical evaluation for symptoms and laboratory evaluation for TSH, FT4, morning cortisol, plasma glucose, and serum electrolytes |
Additional Tests | |
Hypothyroidism | Anti-TPO (antibody) |
Hyperthyroidism | TSH, FT4, T3 every 2–3 weeks, TRAb |
Low Cortisol | ACTH, ACTH stimulation test adrenal CT (in case of suspected primary adrenal insufficiency) |
Hypophysitis | ACTH, LH, FSH, and testosterone in male or estradiol in female pituitary MRI |
Hyperglycemia | Arterial blood gas analysis for metabolic acidosis, urine for ketone body. Autoantibodies such as GAD Islet autoantibodies, insulin autoantibodies, anti-ZnT8 (zinc transporter), serum fasting, and post-mixed meal C-peptide level |
ICPI: Immune checkpoint inhibitor, TSH: Thyroid-stimulating hormone, FT4: Free thyroxine, T3: Triiodothyronine, ACTH: Adrenocorticotropic hormone, CT: Computed tomography, FSH: Follicle-stimulating hormone, LH: Luteinizing hormone, MRI: Magnetic resonance imaging, TRAb: TSH receptor antibody, GAD: Glutamic acid decarboxylase, Anti-TPO: Anti-thyroid peroxidase
CONCLUSION
ICPI therapy has transformed cancer treatment, but it comes with a range of irAEs that differ significantly from the side effects of traditional radiation therapy, chemotherapy, and other cancer treatments. Common irAEs include ICPI-induced thyroid and pituitary dysfunction, while ICPI-related DM, PAI, and other rare endocrinopathies can also occur. If these endocrinopathies are not promptly identified and treated, they can be life-threatening. Educating patients, their family members, and caregivers about the signs and symptoms of ICPI-related endocrinopathies is crucial. Ideally, managing these cases should involve both oncology and endocrinology collaboration. Several challenges remain while diagnosing and managing irEEs, including identifying potential risk factors which could influence ICPI therapy selection, clinical monitoring, and screening approaches. Although hormone deficiencies due to ICPI-related endocrinopathies may be permanent, hormone replacement therapy is effective in most cases.
Ethical approval
The Institutional Review Board approval is not required.
Declaration of patient consent
Patient’s consent was not required as there are no patients in this study.
Conflicts of interest
There are no conflicts of interest.
Use of artificial intelligence (AI)-assisted technology for manuscript preparation
The authors confirm that there was no use of artificial intelligence (AI)-assisted technology for assisting in the writing or editing of the manuscript, and no images were manipulated using AI.
Financial support and sponsorship
Nil.
References
- Insights into the host contribution of endocrine associated immune-related adverse events to immune checkpoint inhibition therapy. Front Oncol. 2022;12:894015.
- [CrossRef] [PubMed] [Google Scholar]
- Differences in CD80 and CD86 transendocytosis reveal CD86 as a key target for CTLA-4 immune regulation. Nat Immunol. 2022;23:1365-78.
- [CrossRef] [PubMed] [Google Scholar]
- CTLA-4 controls follicular helper T-cell differentiation by regulating the strength of CD28 engagement. Proc Natl Acad Sci U S A. 2015;112:524-9.
- [CrossRef] [PubMed] [Google Scholar]
- PD-1 expression by tumour-associated macrophages inhibits phagocytosis and tumour immunity. Nature. 2017;545:495-9.
- [CrossRef] [PubMed] [Google Scholar]
- PD-1 and PD-L1 in cancer immunotherapy: Clinical implications and future considerations. Hum Vaccin Immunother. 2019;15:1111-22.
- [CrossRef] [PubMed] [Google Scholar]
- Incidence of endocrine dysfunction following the use of different immune checkpoint inhibitor regimens: A systematic review and meta-analysis. JAMA Oncol. 2018;4:173-82.
- [CrossRef] [PubMed] [Google Scholar]
- PD-1 inhibitor immune-related adverse events in patients with preexisting endocrine autoimmunity. J Clin Endocrinol Metab. 2018;103:3589-92.
- [CrossRef] [PubMed] [Google Scholar]
- Clinical presentation of immune-related endocrine adverse events during immune checkpoint inhibitor treatment. Cancers (Basel). 2022;14:2687.
- [CrossRef] [PubMed] [Google Scholar]
- Neutrophil-to-lymphocyte ratio predicts development of immune-related adverse events and outcomes from immune checkpoint blockade: A case-control study. Cancers (Basel). 2021;13:1308.
- [CrossRef] [PubMed] [Google Scholar]
- Spectrum of immune checkpoint inhibitors-induced endocrinopathies in cancer patients: A scoping review of case reports. Clin Diabetes Endocrinol. 2019;5:1.
- [CrossRef] [PubMed] [Google Scholar]
- Endocrine toxicities of immune checkpoint inhibitors. Nat Rev Endocrinol. 2021;17:389-99.
- [CrossRef] [PubMed] [Google Scholar]
- Endocrinopathies with use of cancer immunotherapies. Clin Endocrinol (Oxf). 2018;88:327-32.
- [CrossRef] [PubMed] [Google Scholar]
- Thyroid dysfunctions secondary to cancer immunotherapy. J Endocrinol Invest. 2018;41:625-38.
- [CrossRef] [PubMed] [Google Scholar]
- Management of endocrine disease: Immune check point inhibitors-induced hypophysitis. Eur J Endocrinol. 2019;181:R107-18.
- [CrossRef] [PubMed] [Google Scholar]
- Endocrine toxicity and outcomes in patients with metastatic malignancies treated with immune checkpoint inhibitors. J Endocr Soc. 2021;5:bvab100.
- [CrossRef] [PubMed] [Google Scholar]
- Thyroid abnormalities following the use of cytotoxic T-lymphocyte antigen-4 and programmed death receptor protein-1 inhibitors in the treatment of melanoma. Clin Endocrinol (Oxf). 2017;86:614-20.
- [CrossRef] [PubMed] [Google Scholar]
- Characterization of thyroid disorders in patients receiving immune checkpoint inhibition therapy. Cancer Immunol Res. 2017;5:1133-40.
- [CrossRef] [PubMed] [Google Scholar]
- Incidence of thyroid-related adverse events in melanoma patients treated with pembrolizumab. J Clin Endocrinol Metab. 2016;101:4431-9.
- [CrossRef] [PubMed] [Google Scholar]
- Characterization and implications of thyroid dysfunction induced by immune checkpoint inhibitors in real-life clinical practice: A long-term prospective study from a referral institution. J Endocrinol Invest. 2018;41:549-56.
- [CrossRef] [PubMed] [Google Scholar]
- Endocrine toxicity of cancer immunotherapy targeting immune checkpoints. Endocr Rev. 2019;40:17-65.
- [CrossRef] [PubMed] [Google Scholar]
- Endocrine sequelae of immune checkpoint inhibitors. Hormones (Athens). 2017;16:341-50.
- [CrossRef] [PubMed] [Google Scholar]
- Endocrinopathies associated with immune checkpoint inhibitor cancer treatment: A review. J Clin Med. 2020;9:2033.
- [CrossRef] [PubMed] [Google Scholar]
- Nivolumab induced myxedema crisis. J Immunother Cancer. 2017;5:13.
- [CrossRef] [PubMed] [Google Scholar]
- A novel melanoma therapy stirs up a storm: Ipilimumab-induced thyrotoxicosis. Endocrinol Diabetes Metab Case Rep. 2015;2015:140092.
- [CrossRef] [PubMed] [Google Scholar]
- Potential risk factors for nivolumab-induced thyroid dysfunction. In Vivo. 2017;31:1225-8.
- [CrossRef] [Google Scholar]
- Predictive and sensitive biomarkers for thyroid dysfunctions during treatment with immune-checkpoint inhibitors. Cancer Sci. 2020;111:1468-77.
- [CrossRef] [PubMed] [Google Scholar]
- Thyroid disorders in programmed death 1 inhibitor-treated patients: Is previous therapy with tyrosine kinase inhibitors a predisposing factor? Clin Endocrinol (Oxf). 2020;92:258-65.
- [CrossRef] [PubMed] [Google Scholar]
- Ipilimumab-induced orbital inflammation resembling Graves disease with subsequent development of systemic hyperthyroidism from CTLA-4 receptor suppression. Ophthalmic Plast Reconstr Surg. 2014;30:83.
- [CrossRef] [PubMed] [Google Scholar]
- Rapid development of Graves' ophthalmopathy after treatment with ipilimumab and recurrence with pembrolizumab in a patient with previously treated Graves' disease. J Oncol Pract. 2018;14:747-9.
- [CrossRef] [PubMed] [Google Scholar]
- Management of endocrine immune-related adverse events of immune checkpoint inhibitors: An updated review. Endocr Connect. 2020;9:R207-28.
- [CrossRef] [PubMed] [Google Scholar]
- Updates in endocrine immune-related adverse events in oncology immunotherapy. Acta Endocrinol (Buchar). 2021;17:286-9.
- [CrossRef] [PubMed] [Google Scholar]
- Clinical practice guidelines for hypothyroidism in adults: Cosponsored by the American Association of Clinical Endocrinologists and the American Thyroid Association. Endocr Pract. 2012;18:988-1028.
- [CrossRef] [PubMed] [Google Scholar]
- Managing ipilimumab-induced hypophysitis: Challenges and current therapeutic strategies. Cancer Manag Res. 2020;12:9551-61.
- [CrossRef] [PubMed] [Google Scholar]
- Ipilimumab-induced hypophysitis: A detailed longitudinal analysis in a large cohort of patients with metastatic melanoma. J Clin Endocrinol Metab. 2014;99:4078-85.
- [CrossRef] [PubMed] [Google Scholar]
- Immune checkpoint inhibitor-induced hypophysitis and patterns of loss of pituitary function. Front Oncol. 2022;12:836859.
- [CrossRef] [PubMed] [Google Scholar]
- Anti-pituitary antibodies and susceptible human leukocyte antigen alleles as predictive biomarkers for pituitary dysfunction induced by immune checkpoint inhibitors. J Immunother Cancer. 2021;9:e002493.
- [CrossRef] [PubMed] [Google Scholar]
- Endocrine-related adverse events following ipilimumab in patients with advanced melanoma: A comprehensive retrospective review from a single institution. Endocr Relat Cancer. 2014;21:371-81.
- [CrossRef] [PubMed] [Google Scholar]
- Hormonal replacement in hypopituitarism in adults: An endocrine society clinical practice guideline. J Clin Endocrinol Metab. 2016;101:3888-921.
- [CrossRef] [PubMed] [Google Scholar]
- Diagnosis and management of adrenal insufficiency. Lancet Diabetes Endocrinol. 2015;3:216-26.
- [CrossRef] [PubMed] [Google Scholar]
- Evaluation and treatment of adult growth hormone deficiency: An Endocrine Society clinical practice guideline. J Clin Endocrinol Metab. 2011;96:1587-609.
- [CrossRef] [PubMed] [Google Scholar]
- Anti-CTLA-4 antibody therapy associated autoimmune hypophysitis: Serious immune related adverse events across a spectrum of cancer subtypes. Pituitary. 2010;13:29-38.
- [CrossRef] [PubMed] [Google Scholar]
- Anti-PD-L1 treatment induced central diabetes insipidus. J Clin Endocrinol Metab. 2018;103:365-9.
- [CrossRef] [PubMed] [Google Scholar]
- Immune checkpoint inhibitor-associated pituitary adverse events: an observational, retrospective, disproportionality study. J Endocrinol Invest. 2020;43:1473-83.
- [CrossRef] [PubMed] [Google Scholar]
- Hypophysitis secondary to cytotoxic T-lymphocyte-associated protein 4 blockade: Insights into pathogenesis from an autopsy series. Am J Pathol. 2016;186:3225-35.
- [CrossRef] [PubMed] [Google Scholar]
- Secondary adrenal insufficiency: Recent updates and new directions for diagnosis and management. Endocr Pract. 2022;28:110-7.
- [CrossRef] [PubMed] [Google Scholar]
- Management of immune-related adverse events in patients treated with immune checkpoint inhibitor therapy: American Society of clinical oncology clinical practice guideline. J Clin Oncol. 2018;36:1714-68.
- [CrossRef] [PubMed] [Google Scholar]
- Immune checkpoint inhibitor-induced central diabetes insipidus: Looking for the needle in the haystack or a very rare side-effect to promptly diagnose? Front Oncol. 2022;12:798517.
- [CrossRef] [PubMed] [Google Scholar]
- Endocrine-related adverse events related to immune checkpoint inhibitors: Proposed algorithms for management. Oncologist. 2020;25:290-300.
- [CrossRef] [PubMed] [Google Scholar]
- Management of toxicities from immunotherapy: ESMO Clinical Practice Guidelines for diagnosis, treatment and follow-up. Ann Oncol. 2017;28:v119-42.
- [CrossRef] [PubMed] [Google Scholar]
- Immune checkpoint inhibitor-associated primary adrenal insufficiency: WHO VigiBase report analysis. Oncologist. 2020;25:696-701.
- [CrossRef] [PubMed] [Google Scholar]
- Hyponatraemia secondary to nivolumab-induced primary adrenal failure. Endocrinol Diabetes Metab Case Rep. 2016;2016:16-0108.
- [CrossRef] [PubMed] [Google Scholar]
- Immune checkpoint inhibitor-associated new-onset primary adrenal insufficiency: A retrospective analysis using the FAERS. J Endocrinol Invest. 2022;45:2131-7.
- [CrossRef] [PubMed] [Google Scholar]
- Endocrine complications of immunotherapies: A review. Clin Med (Lond). 2021;21:e212-22.
- [CrossRef] [PubMed] [Google Scholar]
- Diagnosis and treatment of primary adrenal insufficiency: An endocrine society clinical practice guideline. J Clin Endocrinol Metab. 2016;101:364-89.
- [CrossRef] [PubMed] [Google Scholar]
- Collateral damage: Insulin-dependent diabetes induced with checkpoint inhibitors. Diabetes. 2018;67:1471-80.
- [CrossRef] [PubMed] [Google Scholar]
- Immune checkpoint inhibitor diabetes mellitus: A novel form of autoimmune diabetes. Clin Exp Immunol. 2020;200:131-40.
- [CrossRef] [PubMed] [Google Scholar]
- Programmed cell death-1 inhibitor-induced type 1 diabetes mellitus. J Clin Endocrinol Metab. 2018;103:3144-54.
- [CrossRef] [PubMed] [Google Scholar]
- Immune checkpoint inhibitors and type 1 diabetes mellitus: A case report and systematic review. Eur J Endocrinol. 2019;181:363-74.
- [CrossRef] [PubMed] [Google Scholar]
- Nivolumab-induced autoimmune diabetes mellitus presenting as diabetic ketoacidosis in a patient with metastatic lung cancer. J Immunother Cancer. 2017;5:40.
- [CrossRef] [PubMed] [Google Scholar]
- Precipitation of autoimmune diabetes with anti-PD-1 immunotherapy. Diabetes Care. 2015;38:e55-7.
- [CrossRef] [PubMed] [Google Scholar]
- A case of fulminant type 1 diabetes mellitus, with a precipitous decrease in pancreatic volume, induced by nivolumab for malignant melanoma: Analysis of HLA and CTLA-4 polymorphisms. Eur J Dermatol. 2017;27:184-5.
- [CrossRef] [PubMed] [Google Scholar]
- Acute-onset type 1 diabetes mellitus caused by nivolumab in a patient with advanced pulmonary adenocarcinoma. J Diabetes Investig. 2017;8:798-9.
- [CrossRef] [PubMed] [Google Scholar]
- Fulminant type 1 diabetes mellitus with anti-programmed cell death-1 therapy. J Diabetes Investig. 2016;7:915-8.
- [CrossRef] [PubMed] [Google Scholar]
- Aggravation of diabetes, and incompletely deficient insulin secretion in a case with type 1 diabetes-resistant human leukocyte antigen DRB1*15:02 treated with nivolumab. J Diabetes Investig. 2018;9:438-41.
- [CrossRef] [PubMed] [Google Scholar]
- Association of serum anti-GAD antibody and HLA haplotypes with type 1 diabetes mellitus triggered by nivolumab in patients with non-small cell lung cancer. J Thorac Oncol. 2017;12:e41-3.
- [CrossRef] [PubMed] [Google Scholar]
- Combined immune checkpoint inhibitor therapy with nivolumab and ipilimumab causing acute-onset type 1 diabetes mellitus following a single administration: Two case reports. BMC Endocr Disord. 2019;19:144.
- [CrossRef] [PubMed] [Google Scholar]
- 15. Diabetes care in the hospital: Standards of medical care in diabetes-2021. Diabetes Care. 2021;44:S211-20.
- [CrossRef] [PubMed] [Google Scholar]
- Immune checkpoint inhibitor-induced hypoparathyroidism associated with calcium-sensing receptor-activating autoantibodies. J Clin Endocrinol Metab. 2019;104:550-6.
- [CrossRef] [PubMed] [Google Scholar]
- Acute symptomatic hypocalcemia from immune checkpoint therapy-induced hypoparathyroidism. Am J Emerg Med. 2017;35:1039.e5-7.
- [CrossRef] [PubMed] [Google Scholar]
- Hypoparathyroidism: An uncommon complication associated with immune checkpoint inhibitor therapy. Mayo Clin Proc Innov Qual Outcomes. 2020;4:821-5.
- [CrossRef] [PubMed] [Google Scholar]