Translate this page into:
Antibody-drug conjugate review in breast cancer: A targeted approach
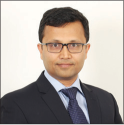
*Corresponding author: Veenoo Agarwal, Department of Medical Oncology, Narayana Superspeciality Hospital, Gurugram, Haryana, India. veenoo@hotmail.com
-
Received: ,
Accepted: ,
How to cite this article: Agarwal V, Mahajan MU, Singh R. Antibody-drug conjugate review in breast cancer: A targeted approach. Int J Mol Immuno Oncol. 2024;9:104-10. doi: 10.25259/IJMIO_30_2024
Abstract
Antibody-drug conjugates (ADCs) provide specificity for cytotoxic drugs as well as monoclonal antibodies (mAbs) and represent an important step in the treatment of breast cancer. This review summarizes the current status, efficacy, safety, and future prospects of ADCs in the treatment of breast cancer. mAbs enable drug delivery by detecting specific antigens on cancer cells. Cytotoxic payloads cause cell death upon internalization. The linker binds the mAb to the payload and influences drug release. All three components together form an ADC. Transtuzumab Emtansine 1 (T-DM1) (Kadcyla) targets human epidermal growth factor receptor 2 (HER2)-positive breast cancer and has shown activity against both metastatic and early disease. Fam-trastuzumab deruxtecan (T-DXd; Enhertu) has shown improved progression-free survival and overall survival for T-DM1 in HER2-positive metastatic breast cancer. Sacituzumab govitecan (SG) targets the Trophoblast Antigen 2 (TROP2) receptor and has been shown to be effective in triple-negative breast cancer and hormone receptor-positive, HER2-negative advanced breast cancer. Fatigue, nausea, and diarrhea are the most common. Some ADCs have their own side effects. T-DXd, which can cause interstitial lung disease, and SG, which increases the risk of diarrhea. Monitoring and controlling these adverse events is important to achieve good patient outcomes. There are ongoing issues with ADC use including drug-related and other issues. The drug-related issues include side effects and ADCs drug resistance. This resistance can be due to a variety of reasons including downregulation of the target antigen, reduced internalization, increased drug efflux, and reduced burden. Strategies to reduce resistance include the use of combination therapies, early detection of resistance, and the development of more effective products. Second-generation ADCs with improved structure and potency are under development. There is ongoing research focusing on novel targets beyond HER2, such as Trop-2 and Claudin-18.2. Non-drug-related issues include tumor heterogeneity, patient selection, manufacturing issues, and increased costs. In conclusion, ADCs are a huge improvement over conventional chemotherapy for breast cancer treatment. The importance and use of ADCs in cancer treatment is bound to increase. The future of ADCs in breast cancer treatment looks promising with ongoing research into new targets, new therapeutic agents, and advances in drug resistance leading to mediated and effective methods.
Keywords
Antibody-drug conjugate
Breast cancer treatment
Novel breast cancer targets
INTRODUCTION
Breast cancer treatment has continually evolved over the years. A deeper understanding of the molecular mechanisms influencing breast cancer, as well as advancements in technology and drug development, have led to a paradigm shift away from conventional chemotherapy and toward targeted treatment. Consequently, targeted therapies have emerged as a cornerstone in the management of breast cancer, offering the potential for improved outcomes and quality of life for patients.
Antibody-drug conjugates (ADCs) represent a novel class of targeted anticancer agents designed to deliver cytotoxic payloads. They use the potency of the cytotoxic payload to enhance antitumor activity and harness the specificity of monoclonal antibodies (mAbs) to reduce systemic toxicity. The ADCs are the next leap forward and help expand the pharmacological toolbox in the battle against cancer, offering a nuanced approach that combines targeted therapy with cytotoxic agents.
The ADCs are the next leap forward and represent a novel family of drugs to expand the pharmacological toolbox in the battle against cancer, offering a nuanced approach that combines targeted therapy with cytotoxic agents.
This review aims to summarize the current status of ADCs in breast cancer treatment, highlighting key clinical trials, efficacy data, safety profiles, ongoing challenges, and opportunities for harnessing the full potential of this innovative class of targeted anticancer therapies.
UNDERSTANDING ADCS
Components of an ADC
An ADC is a molecule made of three components: mAbs, cytotoxic payload, and the linker molecule.
mAbs for targeted delivery
mAbs are the cornerstone of ADCs, serving as precise targeting agents. mAbs are designed to recognize and bind to surface receptors or antigens that are overexpressed (e.g., human epidermal growth factor receptor 2 [HER2]) or unique to cancer cells (such as Trop-2 and Cluster of Differentiation 44 (CD44). By leveraging the high affinity and selectivity of mAbs, ADCs can deliver cytotoxic payloads specifically to cancer cells while sparing healthy tissues. Once attached to the receptor/antigen, the ADC molecule is internalized into the cancer cell.
Cytotoxic payloads: Packing a powerful punch
The cytotoxic payloads, integrated into ADCs, are potent chemotherapeutic agents or biologically active compounds capable of inducing cell death. Examples of cytotoxic payloads used in ADCs for breast cancer include maytansinoids (e.g., Emtansine Derivative 1 (DM1) and Emtansine Derivative 4 (DM4), which are maytansinoids, and Monomethyl Auristatin E (MMAE) and Monomethyl Auristatin F (MMAF), and calicheamicin derivatives. Upon internalization of the ADC into cancer cells following binding to the target antigen, the cytotoxic payload is released, leading to intracellular cytotoxicity and ultimately cell death. The payload’s potency and mechanism of action play a crucial role in determining the therapeutic efficacy of the ADC, emphasizing the importance of selecting appropriate payloads tailored to the specific characteristics of breast cancer subtypes. Precision delivery, internalization, and then activation, maximize therapeutic efficacy while minimizing systemic toxicity. Through strategic selection and optimization of cytotoxic payloads, ADCs have demonstrated remarkable potency in combating breast cancer, offering new avenues for personalized and targeted therapy.
Linkers: The bridge between antibody and payload
As the name implies, the linkers are the molecules that connect the cytotoxic payloads to mAbs. They facilitate the controlled release of the cytotoxic drug into the tumor microenvironment. The linker binds mAbs to the payload, affecting ADC stability and solubility during manufacture and release.
ADC linkers are classified as non-cleavable (like T-DM1) and cleavable (like SYD985). Cleavable linkers release the payload in response to changes in glutathione content, pH, or enzymatic proteolysis thus may lead to higher systemic release. This increases the potential for bystander effect and toxicity. Non-cleavable linkers are stable in both the circulation and the tumor microenvironment. This makes them more specific but may be less effective because of the lack of bystander effect as they must be broken down inside the lysosome following internalization.
Linker design optimization is necessary to provide optimal treatment outcomes, selectivity, and payload release kinetics. Advancements in linker technology have contributed to the clinical success of ADCs in breast cancer by ensuring efficient payload distribution and minimizing premature release and systemic toxicity.
Bystander effect
The cytotoxic effect that the payload or its metabolites have on cell types that are not the ADC’s targets or nearby cells is known as the “bystander effect.” ADC payloads or its metabolites attached with non-cleavable linkers, once internalized and released from the monoclonal antibody component, might be able to pass across the cellular membrane and destroy nearby cells. Cleavable linkers might allow some of the payload to be released into the extracellular space, which would result in direct cytotoxic action.
In theory, the bystander effect increases activity on cancer cells that are not the intended targets. It also decreases activity on cancer cells by lowering the intracellular concentration of the payload and results in a worse side effect profile that may lower the dose that patients can tolerate.
THE IMPACT OF ADCS IN BREAST CANCER
Current approved role in first-line, second-line, and later-line treatment settings
Table 1 shows the current approved role in first-line, second-line, and later-line treatment settings.
First line | Second line | Third line | Fourth line and beyond | |
---|---|---|---|---|
HR-positive HER2-negative HER2 positive | Sacituzumab govitecan Fam-trastuzumab deruxtecan or Ado-trastuzumab emtansine | Ado-trastuzumab emtansine | Margetuximab | |
HER2 low (HER2 IHC 1+or 2+/ISH negative) HR negative HER2 negative (TNBC) | Fam-trastuzumab deruxtecan-Sacituzumab govitecan |
Fam-trastuzumab deruxtecan may be considered in the first-line setting as an option for select patients (i.e., those with rapid progression within 6 months of neoadjuvant or adjuvant therapy [12 months for pertuzumab-containing regimens]). Fam-trastuzumab deruxtecan is associated with interstitial lung disease (ILD)/pneumonitis. Regular monitoring for this serious side effect is recommended. For patients with a history of ILD/pneumonitis, there are no data on managing the safety or toxicity of this drug in a trial. ADC: Antibody-drug conjugate, HER2: Human epidermal growth factor receptor 2, HR: Hormone receptor, TNBC: Triple-negative breast cancer, IHC: Immunohistochemistry, ISH: In Situ Hybridization
Approved ADC drugs for breast cancer
The list of approved drugs has been rising exponentially after the initial approval of 1st drug – T-DM1 (Kadcyla).
T-DM1 (Kadcyla)
T-DM1 is the sentinel drug with HER2 monoclonal antibody trastuzumab backbone. The payload of DM1 molecules (cytotoxic moieties) is linked through a non-reducible thioether linker. This non-cleavable linker results in the release of active DM1 only following the lysosomal proteolytic degradation of the antibody part of T-DM1. DM1-containing metabolites then inhibit microtubule assembly, eventually causing cell death.[1]
In 2013, TDM1 received its first Food and Drug Administration (FDA) approval in metastatic HER2-positive breast cancer following the results of the EMILIA study. EMILIA is a randomized, multicenter, open-label trial (NCT00829166) recruiting 991 patients with HER2-positive, unresectable locally advanced, or metastatic breast cancer. It compared the efficacy of T-DM1 against the then standard-of-care regimen lapatinib + capecitabine.
T-DM1 received its approval in early HER2-positive breast cancer following the results of another phase 3 KATHERINE trial (NCT01772472). KATHERINE was a randomized, multicenter, open-label trial of 1486 patients with HER2-positive, early breast cancer. Patients who had neoadjuvant taxane and trastuzumab-based therapy and did not achieve pathological complete response (pCR), that is, had a residual invasive tumor in the breast and/or axillary lymph nodes, were randomized to receive T-DM1 or standard-of-care trastuzumab. Patients received radiotherapy and/or hormonal therapy concurrent with study treatment as per local guidelines. T-DM1 approval was based on initial results in 2019 showing 3-year invasive disease-free survival (iDFS) with T-DM1, compared with trastuzumab (88.3% vs. 77% trastuzumab). This initial benefit after 3 years[2] has now been shown to be maintained with longer follow-up[3] with 7-year iDFS rates increased from 67.1% with trastuzumab to 80.8% with T-DM1. Importantly, the median overall survival rate (OS) at 7 years rose from 84.4% with trastuzumab to 89.1% with T-DM1. Important efficacy outcomes from the EMILIA and KATHERINE trials[2,4] are listed in Table 2.
Fam-trastuzumab deruxtecan (T-DXd; Enhertu)
T-DXd is a conjugate of a humanized anti-HER2 antibody with a novel DNA topoisomerase I (TOP1) inhibitor using an enzymatically cleavable peptide-based linker. The T-DXd linker reduces hydrophobicity and provides stability in the systemic circulation. In comparison to T-DM1, T-DXd achieves a higher drug-to-antibody ratio with homogeneous conjugation and is highly potent via the bystander antitumor effect.[5]
T-DXd received its initial FDA approval in metastatic HER2-positive breast cancer. The efficacy of T-DXd was evaluated in DESTINY-Breast03 (NCT03529110), a multicenter, open-label, randomized trial that enrolled 524 patients with HER2-positive, unresectable, and/or metastatic breast cancer who received prior trastuzumab and taxane therapy for metastatic disease or developed disease recurrence during or within 6 months of completing adjuvant therapy.
The initial results, reported after the median duration of follow-up of 16 months,[6] highlighted T-DXd’s advantage in progression-free survival (PFS) (not reached vs. 6.8 months). The latest Lancet update[7] after a median follow-up of 28•4 months confirms this PFS benefit (28•8 vs. 6•8 months, hazard ratio (HR) 0.33 [95%confidence interval (CI) 0.26–0.43] nominal P < 0•0001) and goes further by demonstrating a statistically significant improvement in OS.[7]
Important efficacy outcomes from the trial are mentioned in Table 2.
ADC name | Breast cancer subtype | Disease setting | Comparator arm | PFS (months); (HR [95% CI]) | ORR | OS (months); (HR [95% CI]) | iDFS (HR [95% CI]) | DFS |
---|---|---|---|---|---|---|---|---|
T-DM1 | HER2+ | Metastatic | Lapatinib+ Capecitabine | 9.6 versus 6.4 (0.682 [0.548–0.849]) | 43.6% versus 30.8% | 29.9 versus 25.9 (0.75 [0.64–0.88]) | NA | NA |
Adjuvant | Trastuzumab | Data not mature | Data not mature | At 3 years OS data immature | 3-year iDFS 88.3% versus 77% (0.50 [95% CI, 0.39–0.64, P<0.001]) | NA | ||
Median 7-year follow-up OS 89.1% versus 84.4% | 3-year IDFS 88.3% versus 77% trastuzumab) | NA | ||||||
T-DXd | HER2+ | Metastatic | T-DM1 | Median PFS at 12 months 25.1 versus 7.2 with T-DM1 (0.26 [0.20–0.35;P<0.001]) | 79.7% versus 34.2% | OS data immature | At 12 months 75.8–80.7 with T-DXd | |
Median PFS at 28 months 28.8 versus 6.8 (0.33 [0.26–0.43]) | OS data immature |
ADC: Antibody-drug conjugate, HER2: Human epidermal growth factor receptor 2, T-DM1: Tumor drug conjugate 1, PFS: Progression-free survival, OS: Overall survival, iDFS: Invasive disease-free survival, ORR: Objective response rate, CI: Confidence interval, T-DXd: Trastuzumab deruxtecan, DFS: Disease-Free Survival, NS: Not Significant, HR: Hazard Ratio
Sacituzumab govitecan (SG)
SG (TrodelvyTM) is a humanized mAb with the target as the TROP-2 receptor. Sacituzumab has a payload of SN-38 (the active metabolite of irinotecan) chemotherapy drug, which is an irinotecan metabolite. Similar to irinotecan, SN-38 kills cells by inhibiting TOP I. This causes reversible single-strand DNA breaks and prevents DNA re-ligation, which leads to double-strand DNA breakage and cell death.[8,9]
The cleavable linker that attaches the mAbs to SN-38, is a hydrolyzable carbonate moiety. The linker cleavage enables a time-dependent extracellular release of free drugs in the tumor microenvironment. SN-38 has a bystander impact on nearby cells because the payload is membrane-permeable.[8]
The ASCENT-03 trial, a global, open-label, randomized, phase III trial compared the effectiveness of SG to single-agent chemotherapy in heavily pre-treated metastatic triple-negative breast cancer (TNBC) patients. The final results showed that SG significantly improved both PFS (median 4.8 months with SG vs. 1.7 months with physician choice chemotherapy) and OS (median 11.8 months with SG vs. 6.9 months with chemotherapy).[10]
The phase-III TROPiCS-02 trial demonstrated the efficacy of SG, compared with physician’s choice chemotherapy, in patients with endocrine-resistant, chemotherapy-treated hormone receptor-positive (HR+) HER2-negative advanced breast cancer. The study results showed SG clinically meaningful PFS benefit over single-agent chemotherapy (median 5.5 vs. 4.0 months; HR, 0.66; 95% CI, 0.53– 0.83; P = 0.0003).[11] It showed similar improved PFS rates at 6 (46% vs. 30%) and 12 (21% vs. 7%) months.[11] On further follow-up, clinically meaningful OS benefit was reported with a median follow-up of 12.5 months (median 14.4 months vs. 11.2 months; HR-0.79; P = 0.020)[12] and 12.75 months (median, 14.5 vs. 11.2 months; HR, 0.79 [95% CI, 0.65–0.95]; nominal P = 0.01).[13]
SAFETY PROFILE AND MANAGEMENT OF ADVERSE EVENTS ASSOCIATED WITH ADCS
Common side effects of ADCs in breast cancer patients
Extensively pre-treated HR+ HER2-negative breast cancer patients treated with SG showed a reasonable safety profile in the trials. As is typical with cancer treatments, all four ADCs come with a host of common side effects, including lethargy, nausea, and diarrhea. While both T-DM1 and T-DXd can cause similar side effects, T-DXd has a higher risk of pneumonitis and interstitial lung disease (ILD). The serious diarrhea risk with SG is noteworthy; it can be dose-limiting and needs careful control. While other ADCs may not cause neutropenia and hypertension, they come with their own set of specific adverse effects. Common side effects of T-DM1 and T-DXd in breast cancer are listed in Table 3.
Drug | Common adverse events (Any grades) | Common grade ≥3 Adverse events |
---|---|---|
T-DM1 | Thrombocytopenia, elevated transaminases, fatigue, anemia, and nausea | Thrombocytopenia increased aspartate aminotransferase levels and anemia |
T-DXd | Nausea, fatigue, alopecia, vomiting, neutropenia, constipation, anemia, decreased appetite, diarrhea, leukopenia, and thrombocytopenia | Neutropenia, anemia, nausea, leukopenia, lymphopenia, and fatigue |
Sacituzumab | Nausea, diarrhea, fatigue, vomiting, anemia, decreased appetite, alopecia, constipation, rash | Neutropenia, anemia, diarrhea, nausea, fatigue, febrile neutropenia |
T-DM1: Tumor drug conjugate 1, T-DXd: Trastuzumab deruxtecan
Adverse events related to ADCs are mainly due to tumor antigen-specific antibody and cytotoxic payload. The clinical evidence suggests similar rates (2–3%) of reversible cardiac dysfunction related to trastuzumab-related ADCs, including T-DXD, as trastuzumab itself.[7] The cytotoxic payload-related side effects due to T-DXD are manageable and are mainly febrile neutropenia, anemia, nausea, and diarrhea all <10%. T-DXD is associated with 10–15% risk of ILD and needs close monitoring with the appearance of respiratory symptoms, watch oxygen saturation with pulse oximetry and every 8–12 weekly high-resolution computed tomography scan.[14] Grade 3 or more pneumonitis occurs in 2–3% of patients and risk is higher if combined with checkpoint inhibitor therapy or radiation therapy.
There is a need for additional evaluation of dosing in elderly with multiple comorbidities and a need for long-term safety evaluation of ADCs.
RESISTANCE MECHANISMS FOR ADCS
Various Mechanisms related to the resistance of ADCs are as follows:
Downregulation of ADC antigen target
Reduced ADC internalization and trafficking
Increased expression of drug efflux proteins
Reduced action of cytotoxic payload.
To mitigate resistance, the use of ADCs in early lines before target attrition happens, the combination of ADCs with checkpoint inhibitor therapy, and increasing payload potency and therapeutic index would be appropriate strategies.
THE FUTURE OF ADCS IN BREAST CANCER
The advent of ADCs marks a significant stride forward, bolstering therapeutic options for patients grappling with both metastatic and early-stage breast cancer. Nevertheless, we face formidable obstacles as we go forward into the future. Problems such as treatment resistance and tumor heterogeneity, which are associated with patients and cancer, will continue to provide complex hurdles.
Resistance mechanisms to ADCs, as shown by T-DM1 and T-DXd, include complex pathways. Inhibition of HER2, the principal target of T-DM1, is a critical factor that diminishes its effectiveness. The reduced response is also caused by dysregulated endosomal trafficking pathways, signaling through alternative receptor tyrosine kinases, and phosphoinositide 3-kinase (PI3K) signaling. Significant challenges arise when dealing with T-DXd due to driver modifications such as hemizygous deletion of Erythroblastic Oncogene B2 (ERBB2) and decreased HER2 expression.
In this regard, second-generation ADCs stand out as promising candidates, thanks to their improved structures and increased potency. As a promising alternative to previous HER2-targeted treatments, SG shows promise even in individuals who have not responded to these treatments. The therapeutic landscape is opened up to a broader range of patients when we expand our repertoire of targets beyond HER2 to antigens such as Trop-2 and Claudin-18.2.
Targeting the PI3K and fibroblast growth factor receptor pathways are being investigated in HR+ breast cancer, whereas TNBC is seeing a heightened effort to identify effective treatments. New potential targets for targeting the robust resistance mechanisms of TNBC include c-Met, Anexelekto (AXL), folate receptor alpha, and Trop-2.
When anti-programmed cell death-1 ligand 1 treatments are combined with ADCs, a synergistic approach is taken to enhance cancer control. However, even with these advances, opposition remains a major concern. A major challenge is the heterogeneity of breast cancer, which calls for more nuanced methods of patient selection and individualized treatment plans. We are being led toward personalized medicine paradigms by advances in companion diagnostics and tumor profiling, which are crucial instruments in this endeavor.
In addition, there are logistical problems that need to be addressed to make sure that more patients can afford and have access to ADCs. One of these challenges is the complexity of producing the drugs. To ensure that everyone has equal access to these life-changing treatments, we must work together to improve production methods.
Essentially, there are many obstacles on the road ahead, but there is also a great deal of potential. The fight against breast cancer is about to enter a new age if we can decipher the complex processes of resistance, utilize second-generation ADCs to their full potential, and expand our focus to other targets.
CONCLUSION
Traditionally, chemotherapy is a set of toxic yet potent chemicals that are delivered systemically. The chemicals are effective for tumor control but often lead to side effects in healthy tissues. ADCs revolutionize this approach by combining the targeting ability of mAbs to home in the cytotoxic payloads effectively. The antibody acts like a smart missile, attaching to specific antigens on cancer cells, and helping to deliver the deadly payload directly to the tumor site. This targeted strategy minimizes harm to healthy cells, potentially improving tolerability and treatment efficacy.
The story of ADCs in breast cancer goes beyond just targeting cancer cells. Emerging research suggests that ADCs can also stimulate the immune system to recognize and attack tumors, potentially leading to a more durable anti-cancer response. The field of ADC development is constantly evolving and represents a significant advancement in breast cancer treatment. Their targeted approach offers improved tolerability and potentially superior outcomes compared to traditional chemotherapy. With ongoing research exploring novel targets, combination therapies, and overcoming resistance, ADCs are poised to play an even greater role in the fight against breast cancer.
Ethical approval
Institutional Review Board approval is not required.
Declaration of patient consent
Patient’s consent is not required as there are no patients in this study.
Conflicts of interest
There are no conflicts of interest.
Use of artificial intelligence (AI)-assisted technology for manuscript preparation
The authors confirm that there was no use of artificial intelligence (AI)-assisted technology for assisting in the writing or editing of the manuscript and no images were manipulated using AI.
Financial support and sponsorship
Nil.
References
- Trastuzumab emtansine: Mechanisms of action and drug resistance. Breast Cancer Res. 2014;16:209.
- [CrossRef] [PubMed] [Google Scholar]
- Trastuzumab emtansine for residual invasive HER2-positive breast cancer. N Engl J Med. 2019;380:617-28.
- [CrossRef] [PubMed] [Google Scholar]
- Phase III study of adjuvant ado-trastuzumab emtansine vs trastuzumab for residual invasive HER2-positive early breast cancer after neoadjuvant chemotherapy and HER2-targeted therapy: KATHERINE final IDFS and updated OS analysis. Cancer Res. 2024;84(9 Suppl):5-6. Abstract nr GS03-12
- [CrossRef] [Google Scholar]
- Trastuzumab emtansine for HER2-positive advanced breast cancer. N Engl J Med. 2012;367:1783-91.
- [CrossRef] [PubMed] [Google Scholar]
- Trastuzumab deruxtecan: Changing the destiny of HER2 expressing solid tumors. Int J Mol Sci. 2021;22:4774.
- [CrossRef] [PubMed] [Google Scholar]
- Trastuzumab deruxtecan versus trastuzumab emtansine for breast cancer. N Engl J Med. 2022;386:1143-54.
- [CrossRef] [PubMed] [Google Scholar]
- Trastuzumab deruxtecan versus trastuzumab emtansine in patients with HER2-positive metastatic breast cancer: Updated results from DESTINY-Breast03, a randomised, open-label, phase 3 trial. Lancet. 2023;401:105-17.
- [CrossRef] [PubMed] [Google Scholar]
- Mechanism of action of camptothecin. Ann N Y Acad Sci. 2000;922:1-10.
- [CrossRef] [PubMed] [Google Scholar]
- Sacituzumab govitecan, a novel, third-generation, antibody-drug conjugate (ADC) for cancer therapy. Expert Opin Biol Ther. 2020;20:871-85.
- [CrossRef] [PubMed] [Google Scholar]
- Final results from the randomized phase III ASCENT clinical trial in metastatic triple-negative breast cancer and association of outcomes by human epidermal growth factor receptor 2 and trophoblast cell surface antigen 2 expression. J Clin Oncol. 2024;42:1738-44.
- [CrossRef] [PubMed] [Google Scholar]
- Primary results from TROPiCS-02: A randomized phase 3 study of sacituzumab govitecan (SG) versus treatment of physician's choice (TPC) in patients (Pts) with hormone receptor-positive/HER2-negative (HR+/HER2-) advanced breast cancer. J Clin Oncol. 2022;40:LBA1001.
- [CrossRef] [Google Scholar]
- Overall survival with sacituzumab govitecan in hormone receptor-positive and human epidermal growth factor receptor 2-negative metastatic breast cancer (TROPiCS-02): A randomised, open-label, multicentre, phase 3 trial. Lancet. 2023;402:1423-33.
- [CrossRef] [PubMed] [Google Scholar]
- Final overall survival (OS) analysis from the phase 3 TROPiCS-02 study of sacituzumab govitecan (SG) in patients (pts) with hormone receptor-positive/HER2-negative (HR+/HER2-) metastatic breast cancer (mBC) J Clin Oncol. 2023;41(16 Suppl):1003.
- [CrossRef] [Google Scholar]
- Trastuzumab deruxtecan in previously treated HER2-low advanced breast cancer. N Engl J Med. 2022;387:9-20.
- [CrossRef] [PubMed] [Google Scholar]