Translate this page into:
A compendium of population wide DNA methylation profile for oral cancer in India
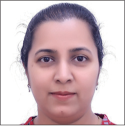
*Corresponding author: Prajakta Zade Fande, Department of Oral, Maxillofacial Pathology and Microbiology, Sharad Pawar Dental College and Hospital, Datta Meghe Institute of Medical Sciences (Deemed To Be University), Wardha, Maharashtra, India. drprajaktafande@gmail.com
-
Received: ,
Accepted: ,
How to cite this article: Fande PZ, Chaudhary MS, Hande AH, Gawande MN, Gadbail AR, Sharma PN, et al. A compendium of population wide DNA methylation profile for oral cancer in India. Int J Mol Immuno Oncol 2021;6(2):82-8.
Abstract
With the emergence of epigenetics, constant attempts are been made to decipher the molecular mechanisms in carcinogenesis. Epigenetic modifications, especially the DNA methylation, have been perceived in oral squamous cell carcinoma (OSCC). The target genes differentially methylated in OSCC still largely remains unknown. There are differences in the molecular alterations in OSCC, regarding geographic location. Therefore, the aim of this review is to present status-quo of existing studies on Indian population to better understand the aberrant patterns of DNA methylation in OSCC that could serve as potential prognostic and diagnostic biomarkers to improve therapy and extend overall survival. The literature was searched using MEDLINE/PubMed, Wiley, Google Scholar, and Science Direct to identify and include most of the relevant articles published from the year 2000 till date in English language. The review would prove to be a valuable resource for population specific investigations and detecting novel biomarkers for OSCC.
Keywords
DNA methylation
Epigenetics
Oral squamous cell carcinoma
Hypermethylation
Hypomethylation
INTRODUCTION
Recently, non-communicable diseases are on surge as they have prevailed beyond our capacity to control it. They are the major cause of preventable deaths and disability. Among these, the most common being cancer has posed a challenge to mankind since its inception. Today, cancer is second major cause of death (first being cardiovascular disease). GLOBOCAN (global cancer incidence, mortality, and prevalence) database, an initiative of International Agency for Research on Cancer is a cancer organization of the World Health Organization; in 2018 reported that worldwide cancer have escalated to 18.1 million new cases and 9.6 million cancer deaths.[1]
They have anticipated that by 2040, the global cancer burden to reach 29.5 million new incident cases and 16.3 million deaths.[2] In the past few years, the role of “epigenetic phenomenon” has emerged significantly. DNA methylation is stable and robust epigenetic marker that often occurs early in cancer development. The genes targeted in oral squamous cell carcinoma (OSCC) still largely remain unknown. So far, no global profile of CpG Island (CGIs) methylation in OSCCs has yet emerged, due to limited samples with few genes being analyzed in the previous studies. Therefore, this comprehensive critical review was conducted to better understand the current status of evidence on DNA methylation patterns in Indian population that may provide insight into the identification of significantly differentially methylated loci/probes (DMPs). This compiled data on target genes affected in specific region of specific population summarizes the significance of epigenetic landscape of OSCC in translational research to achieve early diagnostic and therapeutic landmark. The literature was searched using MEDLINE/PubMed, Wiley, Google Scholar, and Science Direct to identify and include most of the relevant articles published from the year 2000 till date.
ORAL CANCER BURDEN
Oral cancer is a leading public health concern in South Central and Southeast countries of Asia. India has one-third of oral cancer cases of the world and therefore has long been regarded as an epicenter of oral cancer.[3]
It accounts for around 30% of all cancers primarily related to tobacco as reported by the Indian Council of Medical Research. According to GLOBOCAN 2018, new cases of oral cancer in India is estimated to rise from 1.15 million in 2018 to 1.9 million by 2040 and oral cancer deaths is estimated to rise from 0.78 million to 1.33 million by 2040.[2,4] Cancers of lip oral cavity are the leading cause of cancer incidence (16.1%) and mortality (12.3%) in males. The data from National Cancer Registry Programme (Population-Based Cancer Registries) showed highest age-adjusted rate for oral cancer (18.11) in males of Ahmedabad urban, followed by Bhopal (14.2).[5] Among females, it is 4th most common cancer with incidence of 4.8% and mortality 5.9%. Despite easy accessibility to oral cavity, an exponential rise in oral cancer can be attributed to diagnosis at later stages, disparities in access to quality health services and high exposure to risk factors such as tobacco due to easy affordability.[6]
Oral cancer is a multistage, multistep, and multifactorial process. OSCC is the most common oral cavity cancer in Indian subcontinent as compared to developed countries. The risk factors can be broadly classified into two groups – environmental (or modifiable) and genetic (or unmodifiable).[7] The prominent risk factors implicated in oral cancer are tobacco (smoked or smokeless), betel nut/ areca nut, alcohol, human papilloma virus (HPV), exposure to carcinogens (physical, chemical, and biological), diet, and lifestyle. Since long time, environmental and genetic factors were considered to be independent mechanisms, but recent evidence suggests that epigenetics bridges these two factors.[8] However, the genetic and epigenetic mechanisms are distinct but quite closely related. Epigenetic changes depend on modification of DNA unlike genetic changes which depend on DNA sequence change. Genetic changes are stable and irreversible, whereas epigenetic changes are often reversible. The genotype is constant whereas the epigenetic process is dynamic and often changes in response to diseases and environmental factors.[8] Genetic changes usually involve a single gene whereas epigenetics involve more than one gene.[9] Although our epigenetic makeup is more stable during adulthood, it is still thought to be modifiable by lifestyle choices and environmental influence.
EPIGENETIC LANDSCAPE
Conrad Waddington in 1942 first coined the term “Epigenetics.”[10] The word “epi” in Greek means “upon” or “above” or “on top of ” genetics. It turns genes “on” or “off.” These external modifications in DNA does not change its sequence but affects how cells “read” genes.[11-13] Since its recognition, the description modified over time and with consensus in 2008 it is now defined as “stably heritable phenotype resulting from changes in a chromosome without alterations in the DNA sequence.”[14] In addition, epigenetic modifications are transient and potentially reversible. At present, three major epigenetic mechanisms are known – DNA methylation, histone modification, and non-coding RNA -associated gene silencing.
DNA METHYLATION
DNA methylation is perhaps the most common and best studied mechanism. Griffith and Mahler in 1969 first suggested an important biological role of DNA methylation or demethylation.[9] It is covalent addition of a methyl group to the 5-carbon position of cytosine bases that are located 5’ to a guanine base separated by a phosphate molecule in a CpG dinucleotide. These CpG dinucleotides are asymmetrically scattered throughout the genome but are usually found clustered in 0.5–4 kb regions and hence named CGIs.[15] Approximately 50% of genes show presence of CGIs in their promoter regions and methylation mostly occurs in these promoter regions or the first exon sequence.[16] DNA methylation is mediated by different DNA methyltransferases (DNMT), a family of enzymes with potential to transfer methyl group to target DNA by usually involving lysine and arginine residues on histone tails.[10] DNA methylation plays a significant role in regulating gene expression and chromatin architecture.[8] Aberrant methylation patterns have been documented in various cancers. The first being genome-wide hypomethylation of CGIs and the other is hypermethylation of CGIs.
Hypomethylation
Global DNA hypomethylation occurs in repeat sequences, gene deserts, transposons, or CpG dinucleotides located in introns. It causes genome instability, loss of imprinting, and abnormal chromosomal structures. It may also stimulate activation of latent viruses and oncogenes.[16,17]
Hypermethylation
In normal cells with transcriptionally active genes, CGIs are poorly methylated.[17] The highly methylated CGIs in the promoters of genes lead to transcriptional silencing of tumor suppressor genes and thus promotes malignant transformation.[16] Neoplastic cells may simultaneously harbor regional areas of hypermethylation, widespread (global) genomic hypomethylation, and increased DNAmethyltransferase (Dnmt) activity.
DNA METHYLATION PROFILE IN OSCC PATIENTS IN INDIA
OSCC in Indian population has different molecular mechanisms as compared to American and European population, possibly due to differences in environmental and risk factors.[18] The key characteristics of reviewed studies are summarized in Table 1.[19-36] The studies included different sets of population across the states of India. There is heterogeneity among the studies regarding type of sample, sample size, control sampling, socio-economic, and lifestyle (e.g., gutkha, tobacco, and alcohol) characteristics and methylation percent, with less emphasis on data from controls. Over the past two decades, there have been major developments in the methodologies to examine elusive epigenome. With the advent of these molecular techniques, DNA methylation can be examined both at locus-specific context and genome wide. Thirteen studies analyzed methylation patterns using a methylation specific PCR technique (MSP), three studies used methylation sensitive restriction analysis, one study used RT-PCR, and one study used differential methylation hybridization microarray and validated by bisulfite genome sequencing. It is evident from our review that initial studies emphasized more on locus-specific methylation analysis whereas with the evolving molecular techniques, the recent studies focused more on genome wide methylation enabling identification of various differentially methylated sites and genes [Table 2]. For methylation analysis, majority of the studies used biopsy-confirmed tissue samples. Most of the reviewed studies included paired control samples from the same individual adjacent to the tumor tissue to reduce the potential confounding bias. Notably, saliva,[18] scrape,[19] and blood[18,20-24] were also used for analysis. Recently, the use of whole blood and saliva in methylation studies has become common as potential non-invasive mediums for investigation.
N | Author | Year | Parts of India | Cases | Controls | Sample analyzed | Technique | Loci examined |
---|---|---|---|---|---|---|---|---|
1. | Viswanathan et al.[30] | 2003 | South | 99 51(p15) |
25 A | Tissue | MSRA | p16, p15, hMLH1, MGMT, E-cad |
2. | Kulkarni et al.[19] | 2004 | West | 60 | 60A 20 normal mucosa scraping |
Tissue, Scrape |
MSP | p16, DAPK, MGMT GSTP1 |
3. | Ghosh et al.[31] | 2009 | East | 40 dysplastic lesion 63 HNSCC |
40 A 63 A |
Tissue | MSRA | SH3GL2, p14, p15, p16 |
4. | Kaur et al.[18] | 2010 | North | 92 OSCC | 48 A 30 saliva and sera |
Tissue, Saliva, Sera | QMSP | DCC, EDNRB, p16INK4a and KIF1A |
5. | Alyasiri et al.[21] | 2013 | North | 100 | 100A Blood |
Tissue blood control | MSP | PTEN |
6. | Bhatia et al.[20] | 2014 | North | 76 OSCC 54 premalignant |
16 H | Tissue, blood | MSP | MGMT, p16 |
7. | Asokan et al.[32] | 2014 | South | 10 leukoplakia 10 OSCC |
5 H | Tissue | MSP | p16, p15, hMLH, MGMT, E-cad |
8. | Choudhury et al.[33] | 2015 | NE | 71 | 45 A | Tissue | MSP | p16, DAPK, RASSF1, BRAC1, GSTP1, ECAD, MLH1, MINT1, MINT2 and MINT31 |
9. | Sushma et al.[34] | 2015 | South | 50 | 50 A | Tissue | MSP | PTEN, p16 |
10. | Balasubramanian et al.[35] |
2015 | South | 23 | Tissue | MSRA | BRD7 | |
11. | Krishnan et al.[22] | 2016 | South | 52 OTSCC | 52 A | Tissue and/blood | MSP | Whole genome |
12. | Basu et al.[26] | 2017 | East | 64 WDSCC | 64 A | Tissue | MSP | Whole genome |
13. | Jha et al.[23] | 2017 | North | 40 | 20 H | Blood | MSP | FHIT, P14 |
14. | Bhat et al.[29] | 2017 | South | SCCT | DMH validated by BGS | LRPPRC, RAB6C, ZNF471 | ||
15. | Khongsti et al.[27] | 2018 | NE | 12 | 12 A | Tissue | MSP | Whole genome |
16. | Das et al.[28] | 2019 | West, East | 101 OSCC GB | 101 A | Tissue | MSP | Whole genome |
17. | Khongsti et al.[36] | 2019 | NE | 17 | 17 A (2cm away) | Tissue | RT-PCR | FLT3, EPB41L3, SFN |
18. | Goel et al.[24] | 2020 | North | 70 | 20 H blood | Tissue, blood, serum | MSP | LATS2 |
A: Autologous (control from HNSCC themselves), H: Heterogeneous (control from other individuals), NE: Northeast, MSP: Methylation-specific polymerase chain reaction, QMSP: Real-time quantitative MSP, MSRA: PCR-based methylation-sensitive restriction analysis, Pyro: Pyrosequencing, DMH: Differential methylation hybridization microarray and validated by bisulfite genome sequencing (BGS), OTSCC: Oral tongue squamous cell carcinoma, WDSCC: Well differentiated squamous cell carcinoma, GB: Gingivobuccal sulcus
N | Author (year) | CpG sites/ probes (DMP) | CpG regions/genes (DMR) | Hypermethylated genes | Hypomethylated genes |
---|---|---|---|---|---|
1 | Krishnan et al. (2016)[22] | 485,512 | 27,276 | 21,231 | |
2 | Basu et al. (2017)[26] | 21,810 | 5670 | 16,140 | |
3 | Bhat et al. (2017)[29] | 241 | 116 | ||
4 | Khongsti et al. (2018)[27] | 27,205 | 3811 | 38 | 7 |
5 | Das et al. (2019) [28] | 25,321 | 11,522 | 8501 |
DMP/S: Differentially methylated probes/sites, DMR/G: Differentially methylated regions/genes
It is clearly evident that majority of studies reported hypermethylation of the CpG sites than hypomethylation. Table 3 summarizes data on differentially methylated CpG sites. DNA hypomethylation remains less studied as compared to hypermethylation due to its unclear role in carcinogenesis. DNA hypomethylation in cancer is often seen in arthrobacter luteus repeats, satellite DNAs, and long interspersed nuclear elements, etc., mostly in repetitive regions, randomly spread over the genome.[25] These DNA repeats comprise nearly half of the genome. Therefore, DNA hypomethylation is generally considered a global phenomenon not suitable for use as a biomarker. The differential methylation pattern across the CGIs, shores and shelves suggested different mechanisms of hypo- and hypermethylation in OSCC development.[26,27] Das et al. reported considerable variation in proportion of DMPs across the chromosomes. It was found to be highest for chromosome 8 and lowest for chromosome 16. The proportion of hypermethylated DMPs was higher than hypomethylated DMPs in all chromosomes except chromosome 8 and 21.[28] Some of the main genes that are differentially methylated in OSCC are those involved in diverse pathways such as regulation of the cell cycle, physiological signaling and metabolism, proliferation, DNA repair, and apoptosis.[23,28] The ingenuity pathway analysis performed by Basu et al. showed canonical pathways of IL9 signaling and CTLA4 signaling in cytotoxic T lymphocytes to be significantly enriched.[26] Pathway enrichment analysis by Das et al. identified five significantly enriched KEGG pathways (PPAR signaling, arachidonic acid metabolism, B cell receptor signaling pathway, longevity regulating pathway, and genes associated with acute myeloid leukemia) and 22 GO terms (pathways related to G0 to G1 transition, apoptosis, chemokine-mediated signaling pathway, interferon signaling pathway, and various immune-related processes).[28]
N | Author | Year | Loci examined | Methylation pattern |
---|---|---|---|---|
1. | Viswanathan et al.[30] | 2003 | p16, p15, hMLH1, MGMT, E-cad | Hypermethylated |
2. | Kulkarni et al.[19] | 2004 | p16, DAPK, MGMT | Hypermethylated |
3. | Ghosh et al.[31] | 2009 | SH3GL2, p14, p15, p16 | Hypermethylated |
4. | Kaur et al.[18] | 2010 | DCC, EDNRB, p16INK4a and KIF1A |
Hypermethylated |
5. | Alyasiri et al.[21] | 2013 | PTEN | Hypermethylated |
6. | Bhatia et al.[20] | 2014 | MGMT, p16 | Hypermethylated |
7. | Asokan et al.[32] | 2014 | p16, p15, hMLH, MGMT, E-cad | Hypermethylated |
8. | Choudhury et al.[33] | 2015 | p16, DAPK, ECAD, RASSF1, MINT1, MINT2 and MINT31 | Hypermethylated |
9. | Sushma et al.[34] | 2015 | PTEN, p16 | Hypermethylated |
10. | Balasubramanian et al.[35] | 2015 | BRD7 | Hypermethylated |
11. | Krishnan et al.[22] | 2016 | UBE4B, CCDC13, LRP5L, BCL3, MIR4260, FOXK2, and COL18A1 | Hypermethylated |
GSTM2 | Hypomethylated | |||
12. | Basu et al.[26] | 2017 | LXN, ZNF154, ZNF577, ZSCAN31, CTDSP1, LDLRAD4, and HLA-DPB1 | Hypermethylated |
PTPN22, RUNX1, IL6, CD28, TLR1, CD80, CD22, and TNFa CD86, CTLA4 |
Hypomethylated | |||
13. | Jha et al.[23] | 2017 | FHIT, P14 | Hypermethylated |
14. | Bhat et al.[29] | 2017 | LRPPRC, RAB6C, and ZNF471 | Hypermethylated |
15. | Khongsti et al.[27] | 2018 | ADPRH, AOX1, BVES, C17orf107, C3orf62, CHAD, CKMT2, CLDN11, CPXM1, EPB41L3, FAM184B, FLT3, FUZ, GFRA1, GPR81, HOXA4, HOXB1, KCNC3, KHDRBS2, LRAT, MED12L, MME, NEFH, NELL1, NID2, NDY, NRIP2, RUNDC3B, SLC35F1, SNAP91, SYT9, THSD7A, VSX1, WT1, ZNF154, ZNF583, ZSCAN16 | Hypermethylated |
DAPP1, DNAH1, FCRL3, KRT6A, LAMB3, SFN, TM4SF19, TMEM132B | Hypomethylated | |||
16. | Das et al.[28] | 2019 | ZNF132, ZNF626, ZSCAN18, ZNF844 ZNF829, ZNF880, and ZNF229 SH2D2A, PHYHD1, and IGF2BP2 |
Hypermethylated |
CD274, CD80, CD86, DNMT3B | Hypomethylated | |||
17. | Khongsti et al.[36] | 2019 | FLT3, EPB41L3 | Hypermethylated |
SFN | Hypomethylated | |||
18. | Goel et al.[24] | 2020 | LATS2 | Hypermethylated |
Few loci involved in the cell cycle control (p16, p14), DNA repair (MGMT) and apoptosis (DAPK) have been reported consistently in multiple studies and thus appear to be significant markers of choice for further evaluation. A closer look at the current evidences revealed hypomethylation to occur at promoters of genes mainly involved in immune response pathways that induced an anti-tumor T cell response leading to mobilization of T lymphocytes in the neoplastic environment.[26] Genes encoding T lymphocyte regulation such as CD28, CD80, CD86, ZAP70, PI3 kinase, or the PTPN22 tyrosine phosphatase and CTLA4 involved in negatively regulating cytotoxic T cell signaling, are hypomethylated and overexpressed in the neoplastic environment.[27] Kaur et al. associated p16 hypermethylation with nodal involvement and hence poor outcome.[18] Alyasiri et al. indicated PTEN promoter hypermethylation to be more frequent in poorly differentiated OSCC among the Indian population.[21] LATS2 gene promoter hypermethylation was statistically significant in tobacco chewers and smokers.[24] Comparative analysis with the TCGA-HNSCC data revealed 94.6% similarities by Basu et al. and 80.4% similarities by Das et al.[26,28] Khongsti et al. found approximately 29.54% similarities in hypermethylated genes of promoter region. Among 38 genes hypermethylated in promoter region reported by them, 14 genes were similar to TCGA-HNSCC study.[27] Kaplan–Meier survival analysis of TCGA-HNSCC expression data revealed patients with low expressions of DAPK1, RAB6C, and ZNF471 to have poorer survival than patients with high expression (P = 0.02).[29] The epigenome wide methylation analysis identified several novel genes [Table 4]. The functional significance of these novel genes needs to be characterized.
Interestingly, epigenetic alterations in certain cancer genes can be altered by known drugs. The drugs that are known to induce reversal of expressions of such genes include dasatinib, tamoxifen, aspirin, and calcitriol. CD274, CD80, TET1, DNMT3B, PPARG, and PIK3CD gene expressions were altered by these drugs.[28] Although our review revealed some promising cues, some of the studies were subject to limitations in terms of non-uniformity in sampling technique especially control samples. Many hypermethylated loci were reported but it lacked validation. However, the recent genome-wide methylation studies included the validation cohort. Clonal validation by Basu et al. showed hypermethylation in promoter regions of HLA-DPB1 (12–81%), LHX1 (34–98%), LXN (2–29%), and LDLRAD4 (0–38%), and hypomethylation of PTPN22 (30–86%) in tumors compared to adjacent normal tissues.[26] WT1, a candidate gene selected by Khongsti et al. for validation and confirmation experiment clearly showed a significant difference in levels of DNA methylation between the tumor and adjacent normal tissue.[27]
CONCLUSION
There has been general concensus that DNA hypermethylation and hypomethylation patterns are controlled by specific sets of epigenetic genes acting independently but functions simultaneously in different parts of genome. Whole genome screening has identified DNA hypermethylation in promoter region of tumor suppressor genes and global DNA hypomethylation of oncogenes to play crucial role in OSCC. A deeper insight into methylation status of reported “novel genes” with regard to their functional attributes may significantly help to understand oral carcinogenesis. There is an urgent need of population specific approaches to achieve therapeutic and diagnostic milestones that could be applied across geographic locations with success.
This study is relatively the first-ever to present extensive data of Indian population on differentially methylated genes in OSCC that can serve as novel potential DNA methylation biomarker.
Declaration of patient consent
Patient’s consent not required as there are no patients in this study.
Financial support and sponsorship
Nil.
Conflicts of interest
There are no conflicts of interest.
References
- An overview of cancer registration in India: Present status and future challenges. Oncol J India. 2018;2:86.
- [CrossRef] [Google Scholar]
- Burden of cancer in India: GLOBOCAN 2018 estimates incidence, mortality, prevalence and future projections of cancer in India. J Emerg Technol Innov Res. 2019;6:505-14.
- [Google Scholar]
- Opportunistic screening of oral potentially malignant disorders: A public health need for India. JCO Glob Oncol. 2020;6:688-96.
- [CrossRef] [PubMed] [Google Scholar]
- Global patterns and trends in cancers of the lip, tongue and mouth. Oral Oncol. 2020;102:104551.
- [CrossRef] [PubMed] [Google Scholar]
- Squamous cell carcinoma of buccal mucosa: An analysis of prognostic factors. South Asian J Cancer. 2018;7:49-54.
- [CrossRef] [PubMed] [Google Scholar]
- Challenges of the oral cancer burden in India. J Cancer Epidemiol. 2012;2012:701932.
- [CrossRef] [PubMed] [Google Scholar]
- Cancer scenario in India and its comparison with rest of the world and future perspectives. Indian J Community Health. 2019;31:1-3.
- [Google Scholar]
- Epigenetics: A possible answer to the undeciphered etiopathogenesis and behavior of oral lesions. J Oral Maxillofac Pathol. 2016;20:122.
- [CrossRef] [PubMed] [Google Scholar]
- A brief history of epigenetics. Cold Spring Harb Perspect Biol. 2014;6:a018200.
- [CrossRef] [PubMed] [Google Scholar]
- Epigenetics in oral cancer-neoteric biomarker. J Oral Med Oral Surg Oral Pathol Oral Radiol. 2016;2:62.
- [CrossRef] [Google Scholar]
- Epigenetics in oral squamous cell carcinoma. J Oral Maxillofac Pathol. 2017;21:252.
- [CrossRef] [PubMed] [Google Scholar]
- An operational definition of epigenetics. Genes Dev. 2009;23:781-3.
- [CrossRef] [PubMed] [Google Scholar]
- Epigenetic disregulation in oral cancer. Int J Mol Sci. 2012;13:2331-53.
- [CrossRef] [PubMed] [Google Scholar]
- Epigenetic modifications in head and neck cancer. Biochem Genet. 2020;58:213-44.
- [CrossRef] [PubMed] [Google Scholar]
- Epigenetic modifications and head and neck cancer: Implications for tumor progression and resistance to therapy. Int J Mol Sci. 2017;18:1506.
- [CrossRef] [PubMed] [Google Scholar]
- Promoter hypermethylation in Indian primary oral squamous cell carcinoma. Int J Cancer. 2010;127:2367-73.
- [CrossRef] [PubMed] [Google Scholar]
- Concurrent hypermethylation of multiple regulatory genes in chewing tobacco associated oral squamous cell carcinomas and adjacent normal tissues. Oral Oncol. 2004;40:145-53.
- [CrossRef] [Google Scholar]
- Promoter region hypermethylation and mRNA expression of MGMT and p16 genes in tissue and blood samples of human premalignant oral lesions and oral squamous cell carcinoma. Biomed Res Int. 2014;2014:248419.
- [CrossRef] [PubMed] [Google Scholar]
- Aberrant promoter methylation of PTEN gene among Indian patients with oral squamous cell carcinoma. Int J Biol Markers. 2013;28:298-302.
- [CrossRef] [PubMed] [Google Scholar]
- A minimal DNA methylation signature in oral tongue squamous cell carcinoma links altered methylation with tumor attributes. Mol Cancer Res. 2016;14:805-19.
- [CrossRef] [PubMed] [Google Scholar]
- Promoter hypermethylation of FHIT and P14 genes in OSCC patients among north Indian population. Cancer Ther Oncol Int J. 2017;5:555660.
- [CrossRef] [Google Scholar]
- Promoter hypermethylation of LATS2 gene in oral squamous cell carcinoma (OSCC) among North Indian population. Asian Pac J Cancer Prev. 2020;21:1283-7.
- [CrossRef] [PubMed] [Google Scholar]
- Hypomethylation coordinates antagonistically with hypermethylation in cancer development: A case study of leukemia. Hum Genomics. 2016;10(Suppl 2):18.
- [CrossRef] [PubMed] [Google Scholar]
- Genome-wide DNA methylation profile identified a unique set of differentially methylated immune genes in oral squamous cell carcinoma patients in India. Clin Epigenetics. 2017;9:13.
- [CrossRef] [PubMed] [Google Scholar]
- Whole genome DNA methylation profiling of oral cancer in ethnic population of Meghalaya, North East India reveals novel genes. Genomics. 2018;110:112-23.
- [CrossRef] [PubMed] [Google Scholar]
- Epigenomic dysregulation-mediated alterations of key biological pathways and tumor immune evasion are hallmarks of gingivo-buccal oral cancer. Clin Epigenetics. 2019;11:178.
- [CrossRef] [PubMed] [Google Scholar]
- Gene promoter-associated CpG island hypermethylation in squamous cell carcinoma of the tongue. Virchows Arch. 2017;470:445-54.
- [CrossRef] [PubMed] [Google Scholar]
- Promoter hypermethylation profile of tumor-associated genes p16, p15, hMLH1, MGMT and E-cadherin in oral squamous cell carcinoma. Int J Cancer. 2003;105:41-6.
- [CrossRef] [PubMed] [Google Scholar]
- SH3GL2 and CDKN2A/2B loci are independently altered in early dysplastic lesions of head and neck: Correlation with HPV infection and tobacco habit. J Pathol. 2009;217:408-19.
- [CrossRef] [PubMed] [Google Scholar]
- Promoter hypermethylation profile of tumour suppressor genes in oral leukoplakia and oral squamous cell carcinoma. J Clin Diagn Res. 2014;8:ZC09-12.
- [CrossRef] [PubMed] [Google Scholar]
- Promoter hypermethylation profiling identifies subtypes of head and neck cancer with distinct viral, environmental, genetic and survival characteristics. PLoS One. 2015;10:e0129808.
- [CrossRef] [PubMed] [Google Scholar]
- PTEN and p16 genes as epigenetic biomarkers in oral squamous cell carcinoma (OSCC): A study on South Indian population. Tumour Biol. 2016;37:7625-32.
- [CrossRef] [PubMed] [Google Scholar]
- BRD7 promoter hypermethylation as an indicator of well differentiated oral squamous cell carcinomas. Asian Pac J Cancer Prev. 2015;16:1615-9.
- [CrossRef] [PubMed] [Google Scholar]
- Promoter-associated DNA methylation and expression profiling of genes (FLT 3, EPB41L3 and SFN) in patients with oral squamous cell carcinoma in the Khasi and Jaintia population of Meghalaya, India. Indian J Med Res. 2019;150:584.
- [CrossRef] [PubMed] [Google Scholar]